Letter:
Ostrich-Inspired Soft Robotics: A Flexible Bipedal Manipulator for Aggressive Physical Interaction
Hiromi Mochiyama*, Megu Gunji**, and Ryuma Niiyama***
*University of Tsukuba
1-1-1 Tennodai, Tsukuba, Ibaraki 305-8573, Japan
**Toyo University
1-1-1 Izumino, Itakura-machi, Ora-gun, Gunma 374-0113, Japan
***The University of Tokyo
7-3-1 Hongo, Bunkyo-ku, Tokyo 113-8654, Japan
In this letter, ostrich-inspired soft robotics, an approach to intelligent robots that can achieve dexterous manipulation and locomotion without hesitating to collide with the surrounding environment, is proposed. The rationale behind the approach is described from the history of bio-inspired mechanisms, biology, and the theory of robot control. This letter focuses on the manipulator. The first prototype of an ostrich-inspired manipulator was developed to investigate its feasibility. This prototype is a serial chain of 18 rigid links connected with rotation joints moving in a vertical plane and driven through two asymmetric antagonistic wire systems connected to two levers that are directly operated by a human operator playing the role of the controller. Therefore, this manipulator is a highly underactuated mechanism that is flexible against external forces. The experimental results show that a human operator can control this manipulator so that its tip (i.e., the head) can reach several positions, including an upper position against gravity, indicating the potential of ostrich-inspired manipulators.
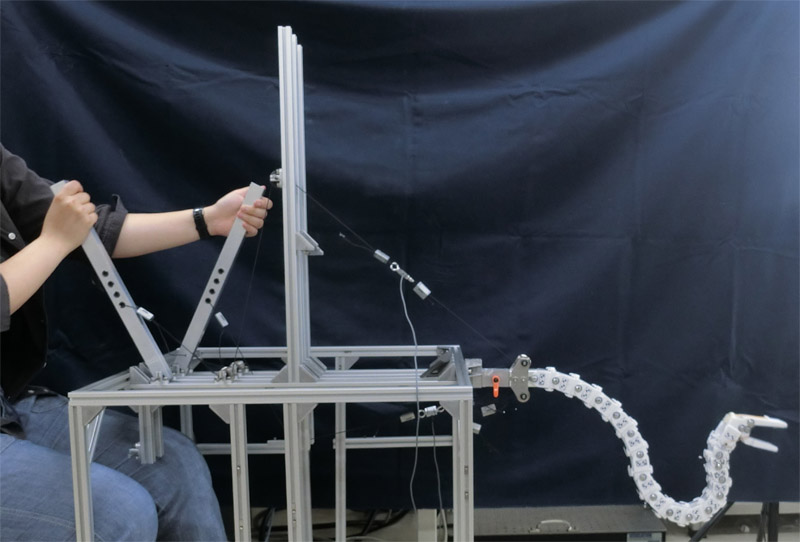
Robostrich, a prototype of an ostrich-inspired manipulator
- [1] S. Olkowicz, M. Kocourek, R. K. Lučan, M. Porteš, W. T. Fitch, S. Herculano-Houzel, and P. Němec, “Birds have primate-like numbers of neurons in the forebrain,” PNAS, Vol.113, No.26, pp. 7255-7260, 2016.
- [2] K. Peng, Y. Feng, G. Zhang, H. Liu, and H. Song, “Anatomical study of the brain of the African ostrich,” Turkish J. of Veterinary and Animal Sciences, Vol.34, No.3, pp. 235-241, 2010.
- [3] K. Hirai, M. Hirose, Y. Haikawa, and T. Takenaka, “The development of Honda humanoid robot,” Proc. 1998 IEEE Int. Conf. on Robotics and Automation (Cat. No.98CH36146), pp. 1321-1326, Vol.2, doi: 10.1109/ROBOT.1998.677288, 1998.
- [4] T. Fukuda, H. Hosokai, and Y. Kondo, “Brachiation type of mobile robot,” Fifth Int. Conf. on Advanced Robotics: Robots in Unstructured Environments, pp. 915-920, Vol.2, doi: 10.1109/ICAR.1991.240556, 1991.
- [5] F. Saito, T. Fukuda, and F. Arai, “Swing and locomotion control for a two-link brachiation robot,” IEEE Control Systems Magazine, Vol.14, No.1, pp. 5-12, doi: 10.1109/37.257888, 1994,
- [6] H. Kajima, Y. Hasegawa, M. Doi, and T. Fukuda, “Energy-based swing-back control for continuous brachiation of a multilocomotion robot,” Int. J. Intell. Syst., Vol.21, No.9, pp. 1025-1043, 2006.
- [7] S. Hirose, “Biologically Inspired Robots: Snake-Like Locomotors and Manipulators,” Oxford Univ Press, 1993.
- [8] M. Mori and S. Hirose, “Development of active cord mechanism ACM-R3 with agile 3D mobility,” Proc. 2001 IEEE/RSJ Int. Conf. on Intelligent Robots and Systems. Expanding the Societal Role of Robotics in the Next Millennium (Cat. No.01CH37180), pp. 1552-1557, Vol.3, doi: 10.1109/IROS.2001.977200, 2001.
- [9] M. Mori and S. Hirose, “Three-dimensional serpentine motion and lateral rolling by active cord mechanism ACM-R3,” IEEE/RSJ Int. Conf. on Intelligent Robots and Systems, pp. 829-834, Vol.1, doi: 10.1109/IRDS.2002.1041493, 2002.
- [10] S. Hirose, T. Kado, and Y. Umeyani, “Tensor Actuated Elastic Manipulator,” Proc. of the Sixth World Congress on Theory of Mechanisms, pp. 978-981, 1983.
- [11] W. McMahan, V. Chitrakaran, M. Csencsits, D. Dawson, I. D. Walker, B. Jones, M. Pritts, D. Dienno, M. Grissom, and C. D. Rahn, “Field Trials and Testing of the OctArm Continuum Manipulator,” Proc. of the 2006 IEEE Int. Conf. on Robotics and Automation (ICRA), pp. 2336-2341, Orlando, FL, May 2006.
- [12] K. Osuka, T. Kinugasa, and T. Ono, “Nonlinear gain scheduling control of legged robot EMU-experimental result,” Proc. of the 1997 IEEE/RSJ Int. Conf. on Intelligent Robot and Systems. Innovative Robotics for Real-World Applications (IROS ’97), pp. V29-V30, Vol.3, doi: 10.1109/IROS.1997.656806, 1997.
- [13] M. Vukobratovic, A. A. Frank, and D. Juricic, “On the Stability of Biped Locomotion,” IEEE Trans. on Biomedical Engineering, Vol.BME-17, No.1, pp. 25-36, doi: 10.1109/TBME.1970.4502681, Jan. 1970.
- [14] A. Takanishi, H.-o. Lim, M. Tsuda, and I. Kato, “Realization of dynamic biped walking stabilized by trunk motion on a sagittally uneven surface,” IEEE Int. Workshop on Intelligent Robots and Systems, Towards a New Frontier of Applications, pp. 323-330, Vol.1, doi: 10.1109/IROS.1990.262408, 1990.
- [15] R. R. Playter and M. H. Raibert, “Control Of A Biped Somersault In 3D,” Proc. of the IEEE/RSJ Int. Conf. on Intelligent Robots and Systems, pp. 582-589, doi: 10.1109/IROS.1992.587396, 1992.
- [16] J. Hurst, “Building Robots That Can Go Where We Go,” IEEE Spectrum, February 2019.
- [17] A. V. Birn-Jeffery, C. M. Hubicki, Y. Blum, D. Renjewski, J. W. Hurst, and M. A. Daley, “Don’t Break a Leg: Running Birds from Quail to Ostrich Prioritise Leg Safety and Economy on Uneven Terrain,” J. of Experimental Biology, Vol.217, pp. 3786-3796, 2014.
- [18] A. Middleditch and J. Oliver, “Functional anatomy of the spine, 2nd ed,” Elsevier Health Sciences, 2005.
- [19] P. Janvier, “Comparative anatomy: all vertebrates do have vertebrae,” Current Biology, Vol.21, No.17, pp. R661-R663, 2011.
- [20] K. F. Liem, W. E. Bemis, W. F. Walker Jr., and L. Grande, “Functional anatomy of the vertebrates – an evolutionary perspective, 3rd ed,” Harcourt college publishers, 2001.
- [21] L. E. Lindell, “The evolution of vertebral number and body size in snakes,” Functional Ecology, Vol.8, pp. 708-719, 1994.
- [22] E. L. Brainerd and S. N. Patek, “Vertebral column morphology, C-start curvature, and the evolution of mechanical defenses in Tetraodontiform fishes,” Copeia, Vol.4, pp. 971-984, 1998.
- [23] F. Galbusera, “The spine: its evolution, function, and shape,” Biomechanics of the spine: basic concepts, spinal disorders and treatments, 1st ed,” F. Galbusera and H.-J. Wilke (Eds.), Academic Press: Cambridge, MA, USA, 2018.
- [24] A. H. J. van der Leeuw, R. G. Bout, and G. A. Zweers, “Evolutionary morphology of the neck system in ratites, fowl and waterfowl,” Neth. J. Zool., Vol.51, No.2, pp. 243-262, 2001.
- [25] Y. Narita and S. Kuratani, “Evolution of the vertebral formulae in mammals: a perspective on developmental constraints,” J. of Experimental Zoology Part B: Molecular and Developmental Evolution, Vol.304, No.2, pp. 91-106, 2005.
- [26] D. M. Wilkinson and G. D. Ruxton, “Understanding selection for long necks in different taxa,” Biological Reviews, Vol.87, No.3, pp. 616-630, 2012.
- [27] M. P. Taylor and M. J. Wedel, “Why sauropods had long necks; and why giraffes have short necks,” Peer J., Vol.1, e36, 2013.
- [28] E. J. Rayfield, “Strain in the ostrich mandible during simulated pecking and validation of specimen-specific finite element models,” J. Anat., Vol.218, No.1, pp. 47-58, 2011.
- [29] S. Kurumaya, H. Nabae, G. Endo, and K. Suzumori, “Design of thin McKibben muscle and multifilament structure,” Sens. Actuators A: Phys., Vol.261, pp. 66-74, 2017.
- [30] N. Yukisawa, Y. Ishi, S. Nishikawa, R. Niiyama, and Y. Kuniyoshi, “Modeling of Extensible Pneumatic Actuator with Bellows (EPAB) for Continuum Arm,” Proc. IEEE Int. Conf. on Robotics and Biomimetics (ROBIO), pp. 2303-2308, 2017.
- [31] G. Oriolo and Y. Nakamura, “Free-joint manipulators: motion control under second-order nonholonomic constraints,” Proc. of IEEE/RSJ Int. Workshop on Intelligent Robots and Systems (IROS ’91), pp. 1248-1253, Vol.3, doi: 10.1109/IROS.1991.174671, 1991.
- [32] T. Suzuki, M. Koinuma, and Y. Nakamura, “Chaos and nonlinear control of a nonholonomic free-joint manipulator,” Proc. of IEEE Int. Conf. on Robotics and Automation, pp. 2668-2675, Vol.3, doi: 10.1109/ROBOT.1996.506565, 1996,
- [33] Y. Nakamura, W. Chung, and O. J. Sordalen, “Design and control of the nonholonomic manipulator,” IEEE Trans. on Robotics and Automation, Vol.17, No.1, pp. 48-59, doi: 10.1109/70.917082. 2001.
- [34] S. Antman, “Nonlinear problems of elasticity, 2nd edition,” Springer, 2004.
- [35] T. Bretl and Z. McCarthy, “Quasi-Static Manipulation of a Kirchhoff Elastic Rod based on a Geometric Analysis of Equilibrium Configurations,” Int. J. of Robotics Research, Vol.33, No.1, pp. 48-68, 2014.
- [36] D. C. Rucker and R. J. Webster III, “Statics and Dynamics of Continuum Robots with General Tendon Routing and External Loading,” IEEE Trans. on Robotics, Vol.27, No.6, pp. 1033-1044, 2011.
- [37] J. Till, C. E. Bryson, S. Chung, A. Orekhov, and D. C. Rucker, “Efficient Computation of Multiple Coupled Cosserat Rod Models for Real-Time Simulation and Control of Parallel Continuum Manipulators,” Proc. of the 2015 IEEE Int. Conf. on Robotics and Automation (ICRA2015), pp. 5067-5074, 2015.
- [38] R. Takano, H. Mochiyama, and N. Takesue, “Real-time Shape Estimation of Kirchhoff Elastic Rod Based on Force/Torque Sensor,” Proc. of the 2017 IEEE Int. Conf. on Robotics and Automation (ICRA2017), pp. 2508-2515, 2017.
- [39] N. Nakagawa and H. Mochiyama, “Real-time Shape Estimation of an Elastic Rod Using a Robot Manipulator Equipped with a Sense of Force,” Proc. of the 2018 IEEE/RSJ Int. Conf. on Intelligent Robots and Systems (IROS2018), pp. 8067-8073, 2018.
- [40] H. Mochiyama, “A Basic Idea of Identifying the Stiffness of an Elastic Rod along its Backbone,” Proc. of the 2018 Int. Symposium on Micro-NanoMechatronics and Human Science (MHS), 2019.
- [41] H. Mochiyama, “The Elastic Rod Approach toward System Theory for Soft Robotics,” Proc. of 21st IFAC World Congress 2020, Vol.53, No.2, pp. 9175-9180, 2020.
- [42] D. Gordon and A. Christian, “Flexibility along the neck of the ostrich (Struthio camelus) and consequences for the reconstruction of dinosaurs with extreme neck length,” J. of Morphology, Vol.268, No.8, pp. 701-714, 2007.
- [43] K. Misu, M. Ikeda, K. Or, M. Ando, M. Gunji, H. Mochiyama, and R. Niiyama, “Robostrich Arm: Wire-driven High-DOF Underactuated Manipulator,” J. Robot. Mechatron., Vol.34, No.2, pp. 328-338, 2022.
This article is published under a Creative Commons Attribution-NoDerivatives 4.0 Internationa License.