Paper:
Comparison of Position Control Performance in Double Air Chambers Pneumatic Artificial Muscle
Naoki Saito*
, Toshiyuki Satoh**
, and Norihiko Saga***

*Akita Prefectural University
84-4 Ebinokuchi, Tsuchiya, Yurihonjo, Akita 015-0055, Japan
**Hirosaki University
3 Bunkyo-cho, Hirosaki, Aomori 036-8561, Japan
***Kwansei Gakuin University
2-1 Gakuen, Sanda, Hyogo 669-1337, Japan
This paper proposes two position control methods for a double air chambers pneumatic artificial muscle (W-PAM) developed to improve the hysteresis characteristics of the rubber-less artificial muscle and confirms their characteristics and usefulness. The proposed control methods are the external pressure-regulated position feedback control and the internal pressure-regulated position feedback control. Of the two air chambers in the W-PAM, the air chamber regulated by the feedback control is different. The steady-state characteristic and frequency characteristics of these controls were compared, and it was experimentally confirmed that the adjusted position feedback control is superior in terms of position control performance. On the other hand, we confirmed that external pressure-regulated position feedback control can express passive stiffness, which is an important characteristic of pneumatic artificial muscles. These results indicate that the W-PAM is a useful actuator for human coexistence systems, because it can express both positional control performance and softness by using different control methods depending on the application.
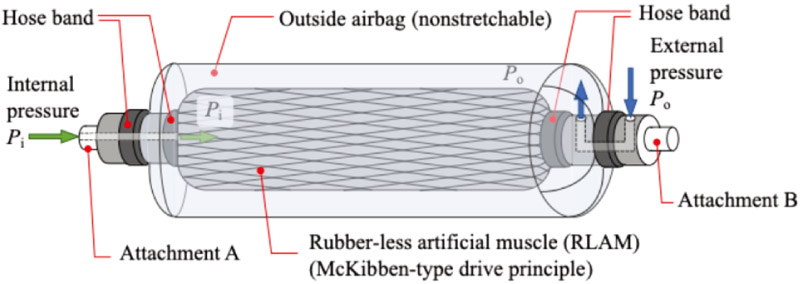
Structure of the W-PAM
- [1] T. Nakamura, “Fluid-driven soft actuators for soft robots,” J. Robot. Mechatron., Vol.36, No.2, pp. 251-259, 2024. https://doi.org/10.20965/jrm.2024.p0251
- [2] P. Polygerinos et al., “Soft robotics: Review of fluid-driven intrinsically soft devices; Manufacturing, sensing, control, and applications in human-robot interaction,” Adv. Eng. Mater., Vol.19, No.12, Article No.1700016, 2017. https://doi.org/10.1002/adem.201700016
- [3] B. Tondu, S. Ippolito, J. Guiochet, and A. Daidie, “A seven-degrees-of-freedom robot-arm driven by pneumatic artificial muscles for humanoid robots,” Int. J. Rob. Res., Vol.24, No.4, pp. 257-274, 2005. https://doi.org/10.1177/0278364905052437
- [4] P. Ohta et al., “Design of a lightweight soft robotic arm using pneumatic artificial muscles and inflatable sleeves,” Soft Robot, Vol.5, No.2, pp. 204-215, 2018. https://doi.org/10.1089/soro.2017.0044
- [5] R. Sawahashi, J. Komatsu, R. Nishihama, M. Okui, and T. Nakamura, “Development of a bimanual wearable force feedback device with pneumatic artificial muscles, MR fluid brakes, and sensibility evaluation based on pushing motion,” J. Robot. Mechatron., Vol.35, No.1, pp. 180-193, 2023. https://doi.org/10.20965/jrm.2023.p0180
- [6] T. Noritsugu, M. Takaiwa, and D. Sasaki, “Development of power assist wear using pneumatic rubber artificial muscles,” J. Robot. Mechatron., Vol.21, No.5, pp. 607-613, 2009. https://doi.org/10.20965/jrm.2009.p0607
- [7] G. Andrikopoulos, G. Nikolakopoulos, and S. Manesis, “A survey on applications of pneumatic artificial muscles,” 19th Mediterranean Conf. Cont. & Autom. (MED), pp. 1439-1446, 2011. https://doi.org/10.1109/med.2011.5982983
- [8] F. Daerden, D. Lefeber, B. Verrelst, and R. van Ham, “Pleated pneumatic artificial muscles: compliant robotic actuators,” Proc. 2001 IEEE/RSJ Int. Conf. Intel. Rob. Sys., Vol.4, pp. 1958-1963, 2001. https://doi.org/10.1109/IROS.2001.976360
- [9] B. Jamil, N. Oh, J.-G. Lee, H. Lee, and H. Rodrigue, “A review and comparison of linear pneumatic artificial muscles,” Int. J. Prec. Eng. Manuf.—Green Tech., Vol.11, No.1, pp. 277-289, 2024. https://doi.org/10.1007/s40684-023-00531-6
- [10] B. Tondu and P. Lopez, “The McKibben muscle and its use in actuating robot-arms showing similarities with human arm behaviour,” Indust. Rob., Vol.24, Issue 6, pp. 432-439, 1997. https://doi.org/10.1108/01439919710192563
- [11] T. Nozaki and T. Noritsugu, “Motion analysis of McKibben type pneumatic rubber artificial muscle with finite element method,” Int. J. Automation Technol., Vol.8, No.2, pp. 147-158, 2014. https://doi.org/10.20965/ijat.2014.p0147
- [12] F. Durante, M. G. Antonelli, P. B. Zobel, and A. Raparelli, “Development of a straight fibers pneumatic muscle,” Int. J. Automation Technol., Vol.12, No.3, pp. 413-423, 2018. https://doi.org/10.20965/ijat.2018.p0413
- [13] A. Kojima, M. Okui, I. Hisamichi, T. Tsuji, and T. Nakamura, “Straight-fiber-type artificial muscle deformation under pressurization,” IEEE Rob. Autom. Letters, Vol.4, No.3, pp. 2592-2598, 2019. https://doi.org/10.1109/LRA.2019.2902016
- [14] T. Hassan, M. Cianchetti, M. Moatamedi, B. Mazzolai, C. Laschi, and P. Dario, “Finite-element modeling and design of a pneumatic braided muscle actuator with multifunctional capabilities,” IEEE/ASME Trans. Mechatron., Vol.24, No.1, pp. 109-119, 2019. https://doi.org/10.1109/TMECH.2018.2877125
- [15] H. Zheng and X. Shen, “Double-acting sleeve muscle actuator for bio-robotic systems,” Actuators, Vol.2, No.4, pp. 129-144, 2013. https://doi.org/10.3390/act2040129
- [16] H. Al-Fahaam, S. Nefti-Meziani, T. Theodoridis, and S. Davis, “The design and mathematical model of a novel variable stiffness extensor-contractor pneumatic artificial muscle,” Soft Robot, Vol.5, No.5, pp. 576-591, 2018. https://doi.org/10.1089/soro.2018.0010
- [17] T. Sato, N. Saito, T. Ogasawara, and T. Sato, “Development of rubberless artificial muscle and fundamental characteristics,” 37th Ann. Conf. IEEE Indust. Elec. Soc., pp. 2124-2129, 2011. https://doi.org/10.1109/IECON.2011.6119636
- [18] N. Saito and T. Satoh, “Structure of a rubberless artificial muscle and evaluation of a lifetime,” 42nd Ann. Conf. IEEE Indust. Elec. Soc., pp. 648-653, 2016. https://doi.org/10.1109/IECON.2016.7793383
- [19] N. Saito, D. Furukawa, T. Satoh, and N. Saga, “Development of semi-crouching assistive device using pneumatic artificial muscle,” J. Robot. Mechatron., Vol.32, No.5, pp. 885-893, 2020. https://doi.org/10.20965/jrm.2020.p0885
- [20] N. Saito, T. Satoh, and N. Saga, “Effects on knee joint force from a body weight load reduction system driven by rubber-less artificial muscle,” Indust. Rob., Vol.46, Issue 5, pp. 642-649, 2019. https://doi.org/10.1108/IR-11-2018-0224
- [21] N. Saito and T. Satoh, “Fundamental development and evaluation of body weight load reduction system driven by rubberless artificial muscle,” 15th Int. Conf. Cont., Autom., Rob. and Vision (ICARCV), pp. 1579-1584, 2018. https://doi.org/10.1109/ICARCV.2018.8581193
- [22] N. Saito, T. Satoh, and N. Saga, “Double air chambers pneumatic artificial muscle and non-hysteresis position control,” Actuators, Vol.13, No.8, Article No.282, 2024. https://doi.org/10.3390/act13080282
- [23] N. Saito, T. Sato, T. Ogasawara, R. Takahashi, and T. Sato, “Mechanical equilibrium model of rubberless artificial muscle and application to position control of antagonistic drive system,” Indust. Rob., Vol.40, Issue 4, pp. 347-354, 2013. https://doi.org/10.1108/01439911311320859
- [24] C.-P. Chou and B. Hannaford, “Measurement and modeling of McKibben pneumatic artificial muscles,” IEEE Trans. Rob. Autom., Vol.12, No.1, pp. 90-102, 1996. https://doi.org/10.1109/70.481753
This article is published under a Creative Commons Attribution-NoDerivatives 4.0 Internationa License.