Paper:
Bio-Inspired Undulatory Locomotion Control Strategy for Novel Soft Robot Based on Auxetic Structures
Shreyas Sharma*
, Takaya Goto*, and Hiroyuki Harada**

*Division of Human Mechanical Systems and Design, Graduate School of Engineering, Hokkaido University
Kita 13, Nishi 9, Kita-ku, Sapporo, Hokkaido 060-0813, Japan
**Division of Mechanical and Aerospace Engineering, Faculty of Engineering, Hokkaido University
Kita 13, Nishi 8, Kita-ku, Sapporo, Hokkaido 060-8628, Japan
This paper presents a novel locomotion soft robot that exploits the properties of auxetic structures to achieve bio-inspired undulatory locomotion. To reduce the dependence on computation and control strategies, we propose to develop a soft structure using a combination of positive Poisson’s ratio lattice structure and negative Poisson’s ratio lattice structure that creates a dorsoventral undulating wave pattern under compressive load. This is combined with a laterally undulating gait pattern exhibited by giant salamanders. The soft structure is actuated with nylon cables attached to servo motors, mimicking muscles. We use finite element analysis (FEA) methods to accurately model the soft structure’s deflection pattern, which is then used to create a control strategy for the robot. We develop a mathematical model and a subsequent gait pattern based on the sequential actuation of the nylon cables. The gait was experimentally tested and further improved with closed-loop error compensation. The research proves linear locomotion is possible through the proposed design with the lowest computational requirements.
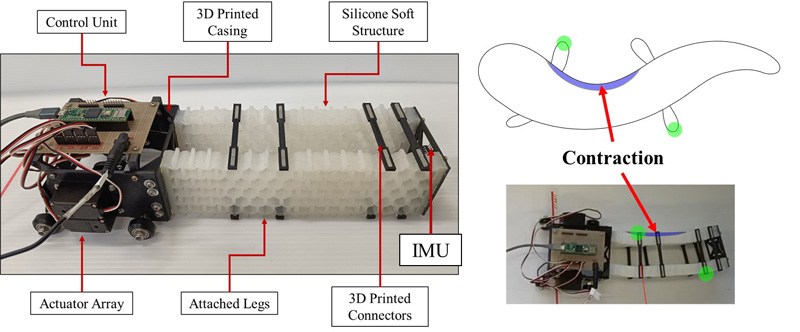
Design of the auxetic soft robot
- [1] D. J. Jurestovsky, L. R. Usher, and H. C. Astley, “Generation of propulsive force via vertical undulations in snakes,” J. of Experimental Biology, Vol.224, No.13, Article No.jeb239020, 2021. https://doi.org/10.1242/jeb.239020
- [2] K. M. Dorgan, C. J. Law, and G. W. Rouse, “Meandering worms: Mechanics of undulatory burrowing in muds,” Proc. of the Royal Society B: Biological Sciences, Vol.280, No.1757, 2013. https://doi.org/10.1098/rspb.2012.2948
- [3] R. E. Shadwick and S. Gemballa, “Structure, kinematics, and muscle dynamics in undulatory swimming,” Fish Physiology, Vol.23, pp. 241-280, 2005. https://doi.org/10.1016/S1546-5098(05)23007-8
- [4] B. C. Jayne, “What defines different modes of snake locomotion?,” Integr. Comp. Biol., Vol.60, No.1, pp. 156-170, 2020. https://doi.org/10.1093/ICB/ICAA017
- [5] O. M. Omisore, S. Han, J. Xiong, H. Li, Z. Li, and L. Wang, “A review on flexible robotic systems for minimally invasive surgery,” IEEE Trans. Syst. Man. Cybern. Syst., Vol.52, No.1, pp. 631-644, 2022. https://doi.org/10.1109/TSMC.2020.3026174
- [6] S. Chen et al., “Soft crawling robots: Design, actuation, and locomotion,” Advanced Materials Technologies, Vol.5, Issue 2, 2020. https://doi.org/10.1002/admt.201900837
- [7] K. Tadakuma, M. Kawakami, and H. Furukawa, “From a deployable soft mechanism inspired by a nemertea proboscis to a robotic blood vessel mechanism,” J. Robot. Mechatron., Vol.34, No.2, pp. 234-239, 2022. https://doi.org/10.20965/jrm.2022.p0234
- [8] Y. Fukuoka, K. Otaka, R. Takeuchi, K. Shigemori, and K. Inoue, “Mechanical designs for field undulatory locomotion by a wheeled snake-like robot with decoupled neural oscillators,” IEEE Trans. on Robotics, Vol.39, No.2, pp. 959-977, 2023. https://doi.org/10.1109/TRO.2022.3226364
- [9] Z. Wolf, A. Jusufi, D. M. Vogt, and G. V Lauder, “Fish-like aquatic propulsion studied using a pneumatically-actuated soft-robotic model,” Bioinspir. Biomim., Vol.15, No.4, Artile No.046008, 2020. https://doi.org/10.1088/1748-3190/ab8d0f
- [10] J. Liu, Y. Tong, and J. Liu, “Review of snake robots in constrained environments,” Rob. Auton. Syst., Vol.141, Artile No.103785, 2021. https://doi.org/10.1016/j.robot.2021.103785
- [11] A. Jusufi, D. M. Vogt, R. J. Wood, and G. V. Lauder, “Undulatory swimming performance and body stiffness modulation in a soft robotic fish-inspired physical model,” Soft Robot., Vol.4, No.3, pp. 202-210, 2017. https://doi.org/10.1089/soro.2016.0053
- [12] C. D. Onal and D. Rus, “Autonomous undulatory serpentine locomotion utilizing body dynamics of a fluidic soft robot,” Bioinspir. Biomim., Vol.8, No.2, Artile No.026003, 2013. https://doi.org/10.1088/1748-3182/8/2/026003
- [13] T. Kano and A. Ishiguro, “Decoding decentralized control mechanism underlying adaptive and versatile locomotion of snakes,” Integr. Comp. Biol., Vol.60, No.1, pp. 232-247, 2020. https://doi.org/10.1093/icb/icaa014
- [14] R. D. Maladen, Y. Ding, C. Li, and D. I. Goldman, “Undulatory swimming in sand: Subsurface locomotion of the sandfish lizard,” Science, Vol.325, No.5938, pp. 314-318, 2009. https://doi.org/10.1126/science.1172490
- [15] R. F. Chapman, “The insects,” Cambridge University Press, 1998. https://doi.org/10.1017/CBO9780511818202
- [16] K. Karakasiliotis, N. Schilling, J. M. Cabelguen, and A. J. Ijspeert, “Where are we in understanding salamander locomotion: Biological and robotic perspectives on kinematics,” Biol. Cybern., Vol.107, No.5, pp. 529-544, 2013. https://doi.org/10.1007/s00422-012-0540-4
- [17] A. Bicanski et al., “Decoding the mechanisms of gait generation in salamanders by combining neurobiology, modeling and robotics,” Biol. Cybern., Vol.107, No.5, pp. 545-564, 2013. https://doi.org/10.1007/S00422-012-0543-1
- [18] R. Matsumoto, S. Fujiwara, and S. E. Evans, “The anatomy and feeding mechanism of the Japanese giant salamander (Andrias japonicus),” J. Anat., Vol.244, No.5, pp. 679-707, 2024. https://doi.org/10.1111/JOA.14004
- [19] M. Mir, M. N. Ali, J. Sami, and U. Ansari, “Review of mechanics and applications of auxetic structures,” Advances in Materials Science and Engineering, Vol.2014, No.1, 2014. https://doi.org/10.1155/2014/753496
- [20] M. B. Francisco, J. L. J. Pereira, G. A. Oliver, L. R. Roque da Silva, S. S. Cunha, and G. F. Gomes, “A review on the energy absorption response and structural applications of auxetic structures,” Mechanics of Advanced Materials and Structures, Vol.29, No.27, pp. 5823-5842, 2022. https://doi.org/10.1080/15376494.2021.1966143
- [21] D. A. Paley and N. M. Wereley, “Bioinspired sensing, actuation, and control in underwater soft robotic systems,” Springer, 2020. https://doi.org/10.1007/978-3-030-50476-2
- [22] W. Zhao, Y. Zhang, and N. Wang, “Soft robotics: Research, challenges, and prospects,” J. Robot. Mechatron., Vol.33, No.1, pp. 45-68, 2021. https://doi.org/10.20965/jrm.2021.p0045
- [23] M. Ishige, T. Umedachi, T. Taniguchi, and Y. Kawahara, “Exploring behaviors of caterpillar-like soft robots with a central pattern generator-based controller and reinforcement learning,” Soft Robot., Vol.6, No.5, pp. 579-594, 2019. https://doi.org/10.1089/soro.2018.0126
This article is published under a Creative Commons Attribution-NoDerivatives 4.0 Internationa License.