Paper:
Gliding Performance of an Insect-Inspired Flapping-Wing Robot
Tatsuya Yamamoto*, Ryusuke Noda**, Hao Liu***, and Toshiyuki Nakata***

*Graduate School of Science and Engineering, Chiba University
1-33 Yayoi-cho, Inage-ku, Chiba, Chiba 263-8522, Japan
**Department of Mechanical Engineering, Tokyo University of Technology
1404-1 Katakuramachi, Hachioji, Tokyo 192-0982, Japan
***Graduate School of Engineering, Chiba University
1-33 Yayoi-cho, Inage-ku, Chiba, Chiba 263-8522, Japan
Flying animals such as insects and birds use wing flapping for flight, occasionally pausing wing motion and transitioning into gliding to conserve energy for propulsion and achieve high flying efficiency. In this study, we have investigated the gliding performance of a gliding model based on a flapping-wing robot developed in a previous study, with the aim of developing a highly efficient flying robot that utilizes bio-inspired intermittent flight. Wind tunnel experiments with a gliding model have shown that the attitude of the wings has a strong influence on gliding performance and that a tail is effective in improving gliding performance. The results of this study provide important insights into the development of flying robots that can travel long distances with high efficiency.
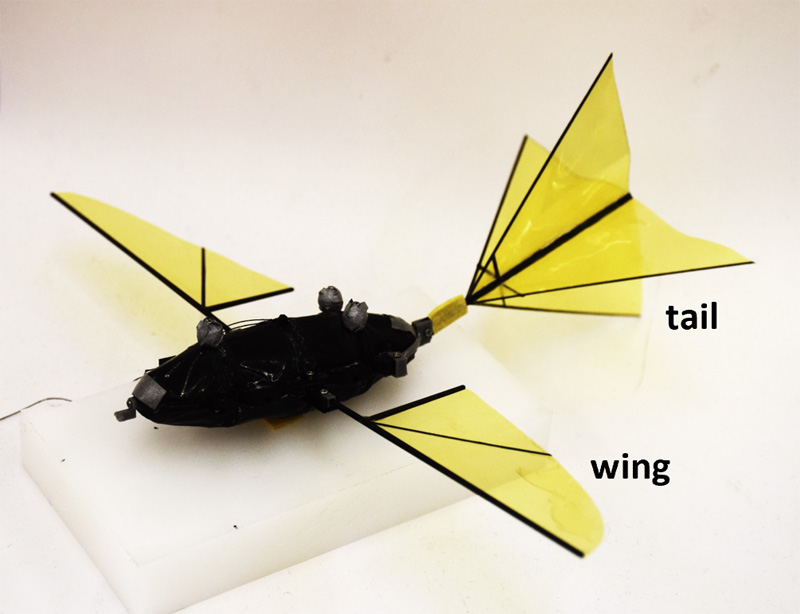
The gliding model based on a flapping-wing robot
- [1] J. W. Chapman, D. R. Reynolds, and K. Wilson, “Long-range seasonal migration in insects: mechanisms, evolutionary drivers and ecological consequences,” Ecol. Lett., Vol.18, No.3, pp. 287-302, 2015. https://doi.org/10.1111/ele.12407
- [2] J. D. Crall, S. Ravi, A. M. Mountcastle, and S. A. Combes, “Bumblebee flight performance in cluttered environments: Effects of obstacle orientation, body size and acceleration,” J. Exp. Biol., Vol.218, Issue 17, pp. 2728-2737, 2015. https://doi.org/10.1242/jeb.121293
- [3] D. Floreano and R. J. Wood, “Science, technology and the future of small autonomous drones,” Nature, Vol.521, No.7553, pp. 460-466, 2015. https://doi.org/10.1038/nature14542
- [4] S. P. Sane, “The aerodynamics of insect flight,” J. Exp. Biol., Vol.206, Issue 23, pp. 4191-4208, 2003. https://doi.org/10.1242/jeb.00663
- [5] B. Tobalske, “Neuromuscular control and kinematics of intermittent flight in the European starling (Sturnus vulgaris),” J. Exp. Biol., Vol.198, Issue 6, pp. 1259-1273, 1995. https://doi.org/10.1242/jeb.198.6.1259
- [6] B. W. Tobalske, “Morphology, velocity, and intermittent flight in birds,” Am. Zool., Vol.41, No.2, pp. 177-187, 2001. https://doi.org/10.1093/icb/41.2.177
- [7] B. W. Tobalske, “Biomechanics of bird flight,” J. Exp. Biol., Vol.210, Issue 18, pp. 3135-3146, 2007. https://doi.org/10.1242/jeb.000273
- [8] J. M. Wakeling and C. P. Ellington, “Dragonfly Flight: I. Gliding Flight and Steady-State Aerodynamic Forces,” J. Exp. Biol., Vol.200, pp. 543-556, 1997. https://doi.org/10.1242/jeb.200.3.543
- [9] J. M. Wakeling and C. P. Ellington, “Dragonfly Flight: II. Velocities, Accelerations and Kinematics of Flapping Flight,” J. Exp. Biol., Vol.200, pp. 557-582, 1997. https://doi.org/10.1242/jeb.200.3.557
- [10] R. J. Bomphrey, T. Nakata, P. Henningsson, and H.-T. Lin, “Flight of the dragonflies and damselflies,” Philos. Trans. R. Soc. Lond. B Biol. Sci., Vol.371, No.1704, 2016. https://doi.org/10.1098/rstb.2015.0389
- [11] P. Paoletti and L. Mahadevan, “Intermittent locomotion as an optimal control strategy,” Proc. Math. Phys. Eng. Sci., Vol.470, No.2164, Article No.20130535, 2014. https://doi.org/10.1098/rspa.2013.0535
- [12] J. M. V. Rayner, P. W. Viscardi, S. Ward, and J. R. Speakman, “Aerodynamics and energetics of intermittent flight in birds,” American Zoologist, Vol.41, No.2, pp. 188-204, 2001. https://doi.org/10.1093/icb/41.2.188
- [13] K. Y. Ma, P. Chirarattananon, S. B. Fuller, and R. J. Wood, “Controlled flight of a biologically inspired, insect-scale robot,” Science, Vol.340, No.6132, pp. 603-607, 2013. https://doi.org/10.1126/science.1231806
- [14] M. Karásek, F. T. Muijres, C. de Wagter, B. D. W. Remes, and G. C. H. E. de Croon, “A tailless aerial robotic flapper reveals that flies use torque coupling in rapid banked turns,” Science, Vol.361, No.6407, pp. 1089-1094, 2018. https://doi.org/10.1126/science.aat0350
- [15] S. Koizumi, T. Nakata, and H. Liu, “Flexibility Effects of a Flapping Mechanism Inspired by Insect Musculoskeletal System on Flight Performance,” Front Bioeng. Biotechnol., Vol.9, Article No.612183, 2021. https://doi.org/10.3389/fbioe.2021.612183
- [16] A. P. Willmott and C. P. Ellington, “The mechanics of flight in the hawkmoth Manduca sexta I. Kinematics of hovering and forward flight,” J. Exp. Biol., Vol.200, pp. 2705-2722, 1997. https://doi.org/10.1242/jeb.200.21.2705
- [17] T. Nakata and H. Liu, “Aerodynamic performance of a hovering hawkmoth with flexible wings: A computational approach,” Proc. Biol. Sci., Vol.279, No.1729, pp. 722-731, 2012. https://doi.org/10.1098/rspb.2011.1023
- [18] B. Etkin, “Dynamics of Atmospheric Flight,” Dover Publication Inc., 2000.
- [19] A. L. Thomas and G. K. Taylor, “Animal flight dynamics I. Stability in gliding flight,” J. Theor. Biol., Vol.212, No.3, pp. 399-424, 2001. https://doi.org/10.1006/jtbi.2001.2387
- [20] A. A. Paranjape, S.-J. Chung, and M. S. Selig, “Flight mechanics of a tailless articulated wing aircraft,” Bioinspir. Biomim., Vol.6, No.2, Article No.026005, 2011. http://doi.org/10.1088/1748-3182/6/2/026005
- [21] D. Lentink et al., “How swifts control their glide performance with morphing wings,” Nature, Vol.446, No.7139, pp. 1082-1085, 2007. https://doi.org/10.1038/nature05733
- [22] C. Harvey, V. B. Baliga, P. Lavoie, and D. L. Altshuler, “Wing morphing allows gulls to modulate static pitch stability during gliding,” J. R. Soc. Interface, Vol.16, No.150, Article No.20180641, 2019. https://doi.org/10.1098/rsif.2018.0641
- [23] C. Harvey, V. B. Baliga, C. D. Goates, D. F. Hunsaker, and D. J. Inman, “Gull-inspired joint-driven wing morphing allows adaptive longitudinal flight control,” J. R. Soc. Interface, Vol.18, No.179, Article No.20210132, 2021. https://doi.org/10.1098/rsif.2021.0132
- [24] C. Harvey, V. B. Baliga, J. C. M. Wong, D. L. Altshuler, and D. J. Inman, “Birds can transition between stable and unstable states via wing morphing,” Nature, Vol.603, pp. 648-653, 2022. https://doi.org/10.1038/s41586-022-04477-8
- [25] C. Harvey and D. J. Inman, “Gull dynamic pitch stability is controlled by wing morphing,” Proc. Natl. Acad. Sci. U.S.A., Vol.119, No.37, Article No.e2204847119, 2022. https://doi.org/10.1073/pnas.2204847119
- [26] E. Chang, L. Y. Matloff, A. K. Stowers, and D. Lentink, “Soft biohybrid morphing wings with feathers underactuated by wrist and finger motion,” Sci. Robot., Vol.5, No.38, 2020. https://doi.org/10.1126/scirobotics.aay1246
- [27] E. Ajanic, M. Feroskhan, S. Mintchev, F. Noca, and D. Floreano, “Bioinspired wing and tail morphing extends drone flight capabilities,” Sci. Robot., Vol.5, No.47, 2020. https://doi.org/10.1126/scirobotics.abc2897
- [28] Y. Murayama, T. Nakata, and H. Liu, “Aerodynamic performance of a bird-inspired morphing tail,” J. Biomech. Sci. Eng., Vol.18, Issue 1, Article No.22-00340, 2023. https://doi.org/10.1299/jbse.22-00340
- [29] B. W. Tobalske and K. P. Dial, “Neuromuscular Control and Kinematics of Intermittent Flight in Budgerigars (Melopsittacus undulatus),” J. Exp. Biol., Vol.187, No.1, pp. 1-18, 1994. https://doi.org/10.1242/jeb.187.1.1
- [30] T. Nakata, R. Noda, S. Kumagai, and H. Liu, “A simulation-based study on longitudinal gust response of flexible flapping wings,” Acta Mech. Sin., Vol.34, No.6, pp. 1048-1060, 2018. https://doi.org/10.1007/s10409-018-0789-5
- [31] J. A. Cheney et al., “Bird wings act as a suspension system that rejects gusts,” Proc. Biol. Sci., Vol.287, No.1937, Article No.20201748, 2020. https://doi.org/10.1098/rspb.2020.1748
- [32] J. P. J. Stevenson, J. A. Cheney, J. R. Usherwood, R. J. Bomphrey, and S. P. Windsor, “Dynamics of hinged wings in strong upward gusts,” Royal Soc. Open Sci., Vol.10, No.5, Article No.221607, 2023. https://doi.org/10.1098/rsos.221607
This article is published under a Creative Commons Attribution-NoDerivatives 4.0 Internationa License.