Paper:
Pedestrian’s Avoidance Behavior Characteristics Against Autonomous Personal Mobility Vehicles for Smooth Avoidance
Ryunosuke Harada*
, Hiroshi Yoshitake*
, and Motoki Shino**
*The University of Tokyo
5-1-5 Kashiwanoha, Kashiwa, Chiba 277-8563, Japan
**Tokyo Institute of Technology
2-12-1 Ookayama, Meguro-ku, Tokyo 152-8550, Japan
Autonomous personal mobility vehicles (PMVs), such as electric wheelchairs, are meant to drive through pedestrian spaces. Cooperative pedestrian avoidance by PMVs is necessary in these spaces to maintain smooth traffic. Previous studies suggested that PMVs can avoid pedestrians on a shorter path without compromising each other’s acceptability. This avoidance can be realized by understanding how pedestrians react to the behavior of PMVs and considering those characteristics in the autonomous navigation of PMVs. In this study, the characteristics of pedestrian’s avoidance behavior were investigated. Experiments were conducted to understand the influence of the parameters of the PMV’s avoidance behavior on pedestrians. Results showed that the angular velocity of the PMV during avoidance affects the pedestrian’s avoidance width and tolerance against the PMV’s behavior. These results suggest that it is possible to avoid pedestrians in smaller avoidance spaces by controlling the angular velocity of the PMV and maintaining smooth traffic.
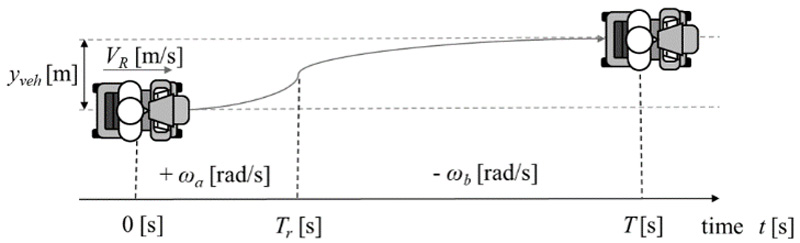
Concept of smooth avoidance method
- [1] A. Brandt, S. Iwarsson, and A. Stahle, “Older People’s Use of Powered Wheelchairs for Activity and Participation,” J. of Rehabilitation Medicine, Vol.36, No.2, pp. 70-77, 2004. https://doi.org/10.1080/16501970310017432
- [2] H. Yoshida, M. Omae, and T. Wada, “Toward Next Active Safety Technology of Intelligent Vehicle,” J. Robot. Mechatron., Vol.27, No.6, pp. 610-616, 2015. https://doi.org/10.20965/jrm.2015.p0610
- [3] H. Darweesh, E. Takeuchi, K. Takeda, Y. Ninomiya, A. Sujiwo, L. Y. Morales, N. Akai, T. Tomizawa, and S. Kato, “Open Source Integrated Planner for Autonomous Navigation in Highly Dynamic Environments,” J. Robot. Mechatron., Vol.29, No.4, pp. 668-684, 2017. https://doi.org/10.20965/jrm.2017.p0668
- [4] T. Suzuki, M. Kitamura, Y. Amano, and N. Kubo, “Autonomous Navigation of a Mobile Robot Based on GNSS/DR Integration in Outdoor Environments,” J. Robot. Mechatron., Vol.26, No.2, pp. 214-224, 2014. https://doi.org/10.20965/jrm.2014.p0214
- [5] K. Mokhtari, A. Ayub, V. Surendran, and A. R. Wagner, “Pedestrian Density Based Path Recognition and Risk Prediction for Autonomous Vehicles,” Proc. of 2020 29th IEEE Int. Conf. on Robot and Human Interactive Communication (RO-MAN), pp. 517-524, 2020. https://doi.org/10.1109/RO-MAN47096.2020.9223554
- [6] A. Rouchitsas and H. Alm, “External Human–Machine Interfaces for Autonomous Vehicle-to-Pedestrian Communication: A Review of Empirical Work,” Frontiers in Psychology, Vol.10, Article No.2757, 2019. https://doi.org/10.3389/fpsyg.2019.02757
- [7] A. Rasouli and K. J. Tsotsos, “Autonomous Vehicles That Interact With Pedestrians: A Survey of Theory and Practice,” IEEE Trans. on Intelligent Transportation Systems, Vol.21, Issue 3, pp. 900-918, 2020. https://doi.org/10.1109/TITS.2019.2901817
- [8] S. Deb, J. L. Strawderman, and W. D. Carruth, “Investigating Pedestrian Suggestions for External Features on Fully Autonomous Vehicles: A Virtual Reality Experiment,” Transportation Research Part F: Traffic Psychology and Behaviour, Vol.59, pp. 135-149, 2018. https://doi.org/10.1016/j.trf.2018.08.016
- [9] Y. Isono, H. Yoshitake, and M. Shino, “Passenger’s Comfort Recognition During Autonomous Navigation of Personal Mobility Vehicles in Crowded Pedestrian Spaces,” Proc. of 17th Int. Joint Conf. on Computer Vision, Imaging and Computer Graphics Theory and Applications (VISIGRAPP’2022), Vol.2, pp. 58-67, 2022. https://doi.org/10.5220/0010849700003124
- [10] C. Liu, Y. Ooeda, and T. Sumi, “A Model for Pedestrian Movement With Obstacle Vision Using Personal Space Concept,” J. Infrastruct. Plan. Manag. D, Vol.64, No.4, pp. 513-524, 2008 (in Japanese). https://doi.org/10.2208/jscejd.64.513
- [11] T. Q. Pham, C. Nakagawa, A. Shintani, and T. Ito, “Evaluation of the Effects of a Personal Mobility Vehicle on Multiple Pedestrians Using Personal Space,” IEEE Trans. on Intelligent Transportation Systems, Vol.16, Issue 4, pp. 2028-2037, 2015. https://doi.org/10.1109/TITS.2014.2388219
- [12] P. Trautman and A. Krause, “Unfreezing the Robot: Navigation in Dense, Interacting Crowds,” Proc. of 2010 IEEE/RSJ Int. Conf. on Intelligent Robots and Systems, pp. 797-803, 2010. https://doi.org/10.1109/IROS.2010.5654369
- [13] K. Miyamoto, H. Yoshioka, N. Watanabe, and Y. Takefuji, “Modeling of Cooperative Behavior Agent Based on Collision Avoidance Decision Process,” Proc. of 2nd Int. Conf. Human-Agent Interaction, pp. 257-260, 2014. https://doi.org/10.1145/2658861.2658936
- [14] Y. Tamura, T. Fukuzawa, and H. Asama, “Smooth Collision Avoidance in Human-Robot Coexisting Environment,” Proc. of 2010 IEEE/RSJ Int. Conf. Intelligent Robots and Systems, pp. 3887-3892, 2010. https://doi.org/10.1109/IROS.2010.5649673
- [15] D. Helbing and P. Molnar, “Social Force Model for Pedestrian Dynamics,” Physical Review E, Vol.51, No.5, pp. 4282-4286, 1995. https://doi.org/10.1103/PhysRevE.51.4282
- [16] Y. Tamura, P. Dai Le, K. Hitomi, N. P. Chandrasiri, T. Bando, A. Yamashita, and H. Asama, “Development of Pedestrian Behavior Model Taking Account of Intention,” Proc. of 2012 IEEE/RSJ Int. Conf. on Intelligent Robots and Systems, pp. 382-387, 2012. https://doi.org/10.1109/IROS.2012.6385599
- [17] S. Thompson, T. Horiuchi, and S. Kagami, “A Probabilistic Model of Human Motion and Navigation Intent for Mobile Robot Path Planning,” Proc. of 2009 4th Int. Conf. on Autonomous Robots and Agents, pp. 663-668, 2009. https://doi.org/10.1109/ICARA.2000.4803931
- [18] H. Kretzschmar, M. Spies, C. Sprunk, and W. Burgard, “Socially Compliant Mobile Robot Navigation via Inverse Reinforcement Learning,” Int. J. of Robotics Research, Vol.35, No.11, pp. 1289-1307, 2016. https://doi.org/10.1177/0278364915619772
- [19] Y. Che, A. M. Okamura, and D. Sadigh, “Efficient and Trustworthy Social Navigation via Explicit and Implicit Robot-Human Communication,” IEEE Trans. on Robotics, Vol.36, No.3, pp. 692-707, 2020. https://doi.org/10.1109/TRO.2020.2964824
- [20] A. Bordallo, F. Previtali, N. Nardelli, and S. Ramamoorthy, “Counterfactual Reasoning About Intent for Interactive Navigation in Dynamic Environments,” Proc. of 2015 IEEE/RSJ Int. Conf. on Intelligent Robots and Systems (IROS), pp. 2943-2950, 2015. https://doi.org/10.1109/IROS.2015.7353783
- [21] K. Li, S. Sun, X. Zhao, J. Wu, and M. Tan, “Inferring User Intent to Interact With a Public Service Robot Using Bimodal Information Analysis,” Advanced Robotics, Vol.33, Nos.7-8, pp. 369-387, 2019. https://doi.org/10.1080/01691864.2019.1599727
- [22] A. D. May, C. Dondrup, and M. Hanheide, “Show Me Your Moves! Conveying Navigation Intention of a Mobile Robot to Humans,” Proc. of 2015 Eur. Conf. on Mobile Robots, 2015. https://doi.org/10.1109/ECMR.2015.7324049
- [23] M. C. Shrestha, T. Onishi, A. Kobayashi, M. Kamezaki, and S. Sugano, “Exploring the Use of Light and Display Indicators for Communicating Directional Intent,” Proc. of 2016 IEEE Int. Conf. on Advanced Intelligent Mechatronics (AIM), pp. 165-1656, 2016. https://doi.org/10.1109/AIM.2016.7577007
- [24] M. C. Shrestha, T. Onishi, A. Kobayashi, M. Kamezaki, and S. Sugano, “Communicating Directional Intent in Robot Navigation using Projection Indicators,” Proc. of 2018 27th IEEE Int. Symp. on Robot and Human Interactive Communication (RO-MAN), pp. 746-751, 2018. https://doi.org/10.1109/ROMAN.2018.8525528
- [25] R. T. Chadalavada, H. Andreasson, M. Schindler, R. Palm, and A. J. Lilienthal, “Bi-Directional Navigation Intent Communication Using Spatial Augmented Reality and Eye-Tracking Glasses for Improved Safety in Human-Robot Interaction,” Robotics and Computer-Integrated Manufacturing, Vol.61, Issue C, Article No.101830, 2020. https://doi.org/10.1016/j.rcim.2019.101830
- [26] R. T. Chadalavada, H. Andreasson, R. Krug, and A. J. Lilienthal, “That’s on my Mind! Robot to Human Intention Communication Through On-Board Projection on Shared Floor Space,” Proc. of 2015 European Conf. on Mobile Robots (ECMR), 2015. https://doi.org/10.1109/ECMR.2015.7403771
- [27] M. Kamezaki, A. Kobayashi, Y. Yokoyama, H. Yanagawa, M. Shrestha, and S. Sugano, “A Preliminary Study of Interactive Navigation Framework with Situation-Adaptive Multimodal Inducement: Pass-By Scenario,” Int. J. of Social Robotics, Vol.12, pp. 567-588, 2020. https://doi.org/10.1007/s12369-019-00574-3
- [28] H. Mori, T. Kurahashi, T. Iwase, T. Machida, A. Kozato, M. Yamashita, and H. Kitaoka, “Modeling of Pedestrian Behavior for Evaluating Safety Systems,” J. of Information Processing Society of Japan, Vol.50, No.12, pp. 3175-3185, 2009 (in Japanese).
- [29] M. Yoda and Y. Shiota, “Mobile Robot’s Passing Motion Algorithm Based on Subjective Evaluation,” Trans. of the JSME, Vol.66, No.650, pp. 156-163, 2000 (in Japanese).
- [30] M. Imanishi and T. Sano, “Analysis of Avoiadnce Behavior From Pedestrian Perspective,” J. of Architecture and Planning, Vol.81, No.719, pp. 57-63, 2016. https://doi.org/10.3130/aija.81.57
This article is published under a Creative Commons Attribution-NoDerivatives 4.0 Internationa License.