Paper:
Ground Adaptability of Crawler Mobile Robots with Sub-Crawler Rotary Joint Compliance
Ayaka Watanabe*1, Tomonori Mitsuhashi*2, Masayuki Okugawa*3, Katsuji Ogane*4, Tetsuya Kimura*5, Tetsuya Kinugasa*6
, and Yoshikazu Ohtsubo*7
*1Sanritz Automation Co., Ltd.
7-47-1-201 Kotobuki-cho, Toyota, Aichi 471-0834, Japan
*2Nagoya Rinkai Rapid Transit Co., Ltd.
1-46 Juichiya, Minato-ku, Nagoya, Aichi 455-0831, Japan
*3Department of Mechanical Engineering, Faculty of Engineering, Aichi Institute of Technology
1247 Yachigusa, Yakusa-cho, Toyota, Aichi 470-0392, Japan
*4Field of Mechanical and System Engineering, Faculty of Engineering, Niigata Institute of Technology
1719 Fujihashi, Kashiwazaki, Niigata 945-1195, Japan
*5Graduate School of Engineering, Nagaoka University of Technology
1603-1 Kamitomioka, Nagaoka, Niigata 940-2188, Japan
*6Department of Mechanical Systems Engineering, Faculty of Engineering, Okayama University of Science
1-1 Ridaicho, Kita-ku, Okayama, Okayama 700-0005, Japan
*7Department of Mechanical Engineering, Faculty of Science and Engineering, Kindai University
3-4-1 Kowakae, Higashi-osaka, Osaka 577-8502, Japan
Remote-controlled mobile robots are expected to be used in difficult- or impossible-to-access environments for inspection workers and responders, such as in investigations and search activities at accident/disaster sites and inspection/investigation work at plants/infrastructure. Among ground mobile robots, crawler mobile robots with sub-crawlers (also known as ground-adaptive crawler robots) excel at in-ground adaptability and stack escape; however, their operators require advanced remote-control technology and experience. Therefore, the introduction of semi-autonomous control to assist the operator is required. In this study, the principle of the pushing-up sequence and the possibility of mobiligence emerging from interaction with obstacles caused by the robot movement were described. In addition, the sub-crawler rotary joint’s compliance, which significantly contributes to ground adaptability, was hypothesized, and a compliance control system design method that uses the sub-crawler constraint angle as a design condition was proposed. It was confirmed that the model robot for the evaluation, which used the proposed method, could adapt to unknown obstacles without measuring their height and shape and traverse them based on experimental results. In addition, based on the numerical calculation results, it was determined that the optimum solution for the restriction angle of the sub-crawler was approximately 35°–50° from the perspective of propulsive force and tumble stability.
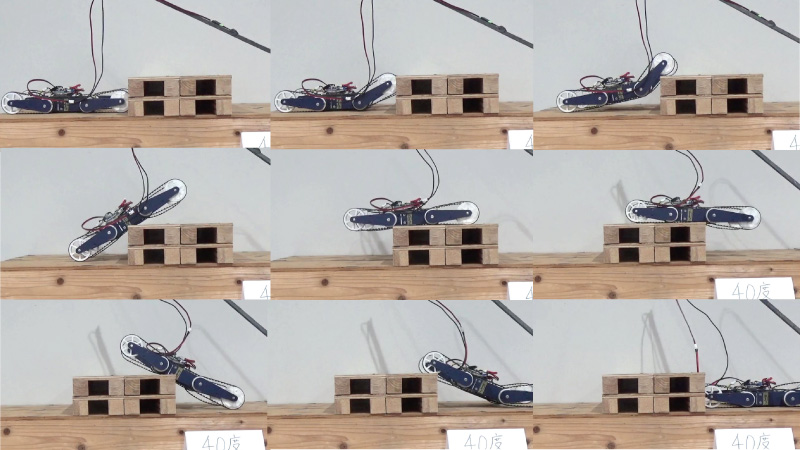
Ground-adaptive crawler robot obstacle traversing
- [1] K. Nagatani, A. Yamasaki, K. Yoshida, T. Yoshida, and E. Koyanagi, “Semi-autonomous traversal on uneven terrain for a tracked vehicle using autonomous control of active flippers,” 2008 IEEE/RSJ Int. Conf. on Intelligent Robots and Systems, pp. 2667-2672, 2008. https://doi.org/10.1109/IROS.2008.4650643
- [2] E. Rohmer et al., “Integration of a sub-crawler’s autonomous control in Quince highly mobile rescue robot,” 2010 IEEE/SICE Int. Symp. on System Integration, pp. 78-83, 2010. https://doi.org/10.1109/SII.2010.5708305
- [3] K. Zimmermann, P. Zuzanek, M. Reinstein, and V. Hlavac, “Adaptive Traversability of unknown complex terrain with obstacles for mobile robots,” 2014 IEEE Int. Conf. on Robotics and Automation (ICRA), pp. 5177-5182, 2014. https://doi.org/10.1109/ICRA.2014.6907619
- [4] M. Mizutani, H. Maruyama, and K. Ito, “Development of autonomous snake-like robot for use in rubble,” 2012 IEEE Int. Symp. on Safety, Security, and Rescue Robotics (SSRR), 2012. https://doi.org/10.1109/SSRR.2012.6523888
- [5] H. Asama et al., “System principle on emergence of mobiligence and its engineering realization,” Proc. 2003 IEEE/RSJ Int. Conf. on Intelligent Robots and Systems (IROS 2003), Vol.2, pp. 1715-1720, 2003. https://doi.org/10.1109/IROS.2003.1248891
- [6] K. Osuka, “Dynamical approach to mobiligence,” J. of the Society of Instrument and Control Engineers, Vol.44, No.9, pp. 640-645, 2005 (in Japanese). https://doi.org/10.11499/sicejl1962.44.640
- [7] Q. Fu and C. Li, “Robotic modelling of snake traversing large, smooth obstacles reveals stability benefits of body compliance,” Royal Society Open Science, Vol.7, No.2, Article No.191192, 2020. https://doi.org/10.1098/rsos.191192
- [8] T. Kinugasa, K. Osuka, R. Hayashi, N. Miyamoto, and K. Yoshida, “Development of a small and lightweight myriapod robot using passive dynamics,” Artificial Life and Robotics, Vol.22, No.4, pp. 429-434, 2017. https://doi.org/10.1007/s10015-017-0378-x
- [9] S. Suzuki, S. Hasegawa, and M. Okugawa, “Remote control system of disaster response robot with passive sub-crawlers considering falling down avoidance,” ROBOMECH J., Vol.1, No.1, Article No.20, 2014. https://doi.org/10.1186/s40648-014-0020-9
- [10] S. Kang, W. Lee, M. Kim, and K. Shin, “ROBHAZ-rescue: Rough-terrain negotiable teleoperated mobile robot for rescue mission,” IEEE Int. Safety, Security and Rescue Rototics, Workshop, pp. 105-110, 2005. https://doi.org/10.1109/SSRR.2005.1501248
- [11] J. Hirasawa and T. Kimura, “Development of stair-climbing mechanism with passive crawlers (Analysis of limitation for crawler rotation angle and test vehicle performance),” Trans. of the JSME, Vol.82, No.834, Article No.15-00357, 2016 (in Japanese). https://doi.org/10.1299/transjsme.15-00357
- [12] H. Miura, A. Watanabe, M. Okugawa, T. Miura, and T. Koganeya, “Plant inspection by using a ground vehicle and an aerial robot: Lessons learned from plant disaster prevention challenge in world robot summit 2018,” Advanced Robotics, Vol.34, No.2, pp. 104-118, 2020. https://doi.org/10.1080/01691864.2019.1690575
- [13] H. Miura et al., “Field experiment report for verification of abandoned lignite mines by robotic exploration system,” J. Robot. Mechatron., Vol.30, No.6, pp. 1004-1013, 2018. https://doi.org/10.20965/jrm.2018.p1004
- [14] H. Miura, A. Watanabe, M. Okugawa, and T. Miura, “Verification and evaluation of robotic inspection of the inside of culvert pipes,” J. Robot. Mechatron., Vol.31, No.6, pp. 794-802, 2019. https://doi.org/10.20965/jrm.2019.p0794
- [15] A. Watanabe et al., “Effect of compliance on ground adaptability of crawler mobile robots with sub-crawlers,” 2020 IEEE/SICE Int. Symp. on System Integration (SII), pp. 1348-1353, 2020. https://doi.org/10.1109/SII46433.2020.9026250
- [16] S. Hirose, “Mechanical design of mobile robot for external environments,” J. of the Robotics Society of Japan, Vol.18, No.7, pp. 904-908, 2000 (in Japanese). https://doi.org/10.7210/jrsj.18.904
- [17] E. Messina and A. Jacoff, “Performance standards for urban search and rescue robots,” Proc. of SPIE6230, Unmanned Systems Technology VIII, Article No.62301V, 2006. https://doi.org/10.1117/12.663320
- [18] G. Gabrielli and T. von Karman, “What price speed?: Specific power required for propulsion of vehicles,” Mechanical Engineering, Vol.72, No.10, pp. 775-781, 1950.
- [19] S. Hirose, H. Tsukagoshi, and K. Yoneda, “Normalized energy stability margin and its contour of walking vehicles on rough terrain,” Proc. of 2001 IEEE Int. Conf. on Robotics and Automation (ICRA), Vol.1, pp. 181-186, 2001. https://doi.org/10.1109/ROBOT.2001.932550
This article is published under a Creative Commons Attribution-NoDerivatives 4.0 Internationa License.