Paper:
Analysis of Separation Efficiency Focusing on Particle Concentration and Size Using a Spiral Microfluidic Device
Mitsuhiro Horade*
, Syunsuke Mukae*, Tasuku Yamawaki*, Masahito Yashima*
, Shuichi Murakami**, and Tsunemasa Saiki***
*Department of Mechanical Systems Engineering, National Defense Academy of Japan
1-10-20 Hashirimizu, Yokosuka, Kanagawa 239-8686, Japan
**Osaka Research Institute of Industrial Science and Technology
2-7-1 Ayumino, Izumi, Osaka 594-1157, Japan
***Hyogo Prefectural Institute of Technology
3-1-12 Yukihira, Suma, Kobe, Hyogo 654-0037, Japan
This study discusses component separation using a microfluidic device. Based on the separation principle, a method was adopted to generate an external force due to centrifugal force in a spirally designed channel. In this study, four types of polystyrene particles with different diameters ranging within 1–45 µm were used, and the separation performance was evaluated for each particle size. The centrifugal force increased as the flow velocity in the channel increased; however, this time, the test was conducted with the flow rate, which is an input parameter fixed at 100 µL/min. The results of the micro-channel observation using a high-speed camera indicated that the particle density might be a factor in the decrease in separation efficiency. Therefore, by conducting tests at three different particle densities, we were able to experimentally investigate the change in separation efficiency based on the particle size and density. In this study, we considered the separation efficiency due to the size and density of the particle diameter along with its application to an onsite-type separation device.
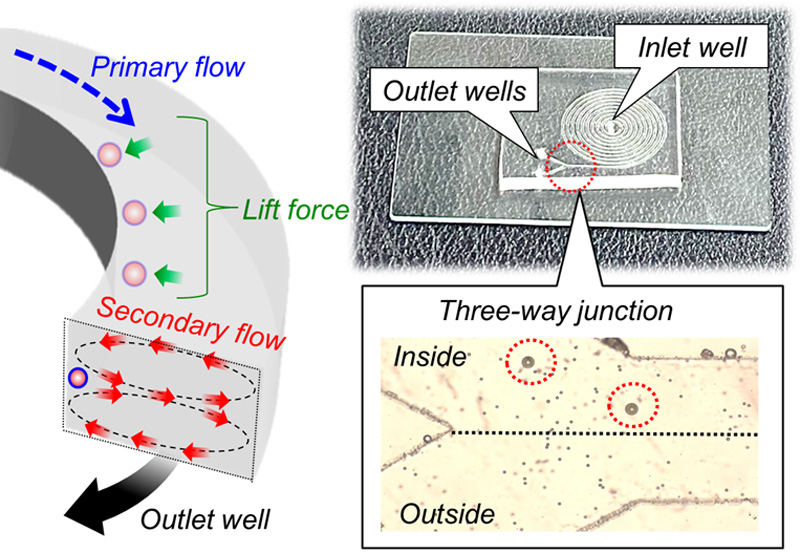
Image of particle sorting using a spiral microfluidic device
- [1] S. Pandey, N. Mehendale, and D. Paul, “Single-Cell Separation,” T. S. Santra and F.-G. Tseng (Eds.), “Handbook of Single-Cell Technologies,” Springer Singapore, pp. 207-234, 2021. https://doi.org/10.1007/978-981-10-8953-4_6
- [2] Y. Deng, Y. Guo, and B. Xu, “Recent Development of Microfluidic Technology for Cell Trapping in Single Cell Analysis: A Review,” Processes, Vol.8, No.10, pp. 1253-1283, 2020. https://doi.org/10.3390/pr8101253
- [3] J. Nilsson, M. Evander, B. Hammarström, and T. Laurell, “Review of cell and particle trapping in microfluidic systems,” Analytica Chimica Acta, Vol.649, Issue 2, pp. 141-157, 2009. https://doi.org/10.1016/j.aca.2009.07.017
- [4] X. He, H. Kimura, and T. Fujii, “A High-Throughput Device for Patterned Differentiation of Embryoid Bodies,” J. Robot. Mechatron., Vol.25, No.4, pp. 623-630, 2013. https://doi.org/10.20965/jrm.2013.p0623
- [5] M. Iwase, M. Yamada, E. Yamada, and M. Seki, “Formation of Cell Aggregates Using Microfabricated Hydrogel Chambers for Assembly into Larger Tissues,” J. Robot. Mechatron., Vol.25, No.4, pp. 682-689, 2013. https://doi.org/10.20965/jrm.2013.p0682
- [6] T. Konishi, Y. Jingu, T. Yoshizawa, M. Irita, T. Suzuki, and M. Hayase, “Mitigation of Channel Clogging in a Microfluidic Device for Capturing Circulating Tumor Cells,” Int. J. Automation Technol., Vol.14, No.1, pp. 109-116, 2020. https://doi.org/10.20965/ijat.2020.p0109
- [7] M. Callewaert, J. O. D. Beeck, K. Maeno, S. Sukas, H. Thienpont, H. Ottevaere, H. Gardeniers, G. Desmeta, and W. D. Malsche, “Integration of uniform porous shell layers in very long pillar array columns using electrochemical anodization for liquid chromatography,” Analyst, Vol.139, No.3, pp. 618-625, 2014. https://doi.org/10.1039/C3AN02023A
- [8] H. M. Ji, V. Samper, Y. Chen, C. K. Heng, T. M. Lim, and L. Yobas, “Silicon-based microfilters for whole blood cell separation,” Biomedical Microdevices, Vol.10, pp. 251-257, 2008. https://doi.org/10.1007/s10544-007-9131-x
- [9] Y. Jiao, Y. He, and F. Jiao, “Two-dimensional Simulation of Motion of Red Blood Cells with Deterministic Lateral Displacement Devices,” Micromachines, Vol.10, No.6, Article No.393, 2019. https://doi.org/10.3390/mi10060393
- [10] T. Robinson, P. Kuhn, K. Eyer, and P. S. Dittrich, “Microfluidic trapping of giant unilamellar vesicles to study transport through a membrane pore,” Biomicrofluidics, Vol.7, Article No.044105, 2013. https://doi.org/10.1063/1.4816712
- [11] Y. Yalikun, Y. Akiyama, T. Hoshino, and K. Morishima, “A Bio-Manipulation Method Based on the Hydrodynamic Force of Multiple Microfluidic Streams,” J. Robot. Mechatron., Vol.25, No.4, pp. 611-618, 2013. https://doi.org/10.20965/jrm.2013.p0611
- [12] T. Takayama, M. Kaneko, and C.-H. D. Tsai, “On-Chip Micro Mixer Driven by Elastic Wall with Virtual Actuator,” Micromachines, Vol.12, No.2, Article No.217, 2021. https://doi.org/10.3390/mi12020217
- [13] G. Chitnis, Z. Ding, C. L. Chang, C. A. Savran, and B. Ziaie, “Laser-treated hydrophobic paper: an inexpensive microfluidic platform,” Lab on a Chip, Vol.11, No.6, pp. 1161-1165, 2011. https://doi.org/10.1039/C0LC00512F
- [14] S. Fruncillo, X. Su, H. Liu, and L. S. Wong, “Lithographic Processes for the Scalable Fabrication of Micro- and Nanostructures for Biochips and Biosensors,” ACS Sensors, Vol.6, No.6, pp. 2002-2024, 2021. https://doi.org/10.1021/acssensors.0c02704
- [15] R. Palankar, N. Medvedev, A. Rong, and M. Delcea, “Fabrication of Quantum Dot Microarrays Using Electron Beam Lithography for Applications in Analyte Sensing and Cellular Dynamics,” ACS Nano, Vol.7, No.5, pp. 4617-4628, 2013. https://doi.org/10.1021/nn401424y
- [16] L. Zhai, M. C. Berg, F. Ç. Cebeci, Y. Kim, J. M. Milwid, M. F. Rubner, and R. E. Cohen, “Patterned superhydrophobic surfaces: toward a synthetic mimic of the Namib Desert beetle,” Nano Letters, Vol.6, No.6, pp. 1213-1217, 2006. https://doi.org/10.1021/nl060644q
- [17] M. Horade, M. Kojima, K. Kamiyama, Y. Mae, and T. Arai, “Development of a novel 2-dimensional micro-heater array device with regional selective heating,” Mech. Eng. Res., Vol.6, No.1, pp. 66-74, 2016. https://doi.org/10.5539/mer.v6n1p66
- [18] Q. Ramadana, V. Samperb, D. P. Poenarc, and C. Yu, “An integrated microfluidic platform for magnetic microbeads separation and confinement,” Biosensors and Bioelectronics, Vol.21, No.9, pp. 1693-1702, 2005. https://doi.org/10.1016/j.bios.2005.08.006
- [19] F. Alnaimat, B. Mathew, and A. H. Alnaqbi, “Modeling a Dielectrophoretic Microfluidic Device with Vertical Interdigitated Transducer Electrodes for Separation of Microparticles Based on Size,” Micromachines, Vol.11, No.6, Article No.563, 2020. https://doi.org/10.3390/mi11060563
- [20] K. Oshiro, Y. Wakizaka, M. Takano, T. Itoi, H. Ohge, K. Koba, K. Yarimizu, S. Fujiyoshi, and F. Maruyama, “Fabrication of a new all-in-one microfluidic dielectrophoresis integrated chip and living cell separation,” Iscience, Vol.25, Issue 2, Article No.103776, 2022. https://doi.org/10.1016/j.isci.2022.103776
- [21] V. Varmazyari, H. Habibiyan, H. Ghafoorifard, M. Ebrahimi, and S. Ghafouri-Fard, “A dielectrophoresis-based microfluidic system having double-sided optimized 3D electrodes for label-free cancer cell separation with preserving cell viability,” Scientific Reports, Vol.12, Article No.12100, 2022. https://doi.org/10.1038/s41598-022-16286-0
- [22] M. Saito, K. Takahashi, Y. Kiriyama, W. V. Espulgar, H. Aso, T. Sekiya, Y. Tanaka, T. Sawazumi, S. Furui, and E. Tamiya, “Centrifugation-controlled thermal convection and its application to rapid microfluidic polymerase chain reaction devices,” Analytical Chemistry, Vol.89, pp. 12797-12804, 2017. https://doi.org/10.1021/acs.analchem.7b03107
- [23] K. Wang, R. Liang, H. Chen, S. Lu, S. Jia, and W. Wang, “A microfluidic immunoassay system on a centrifugal platform,” Sensors and Actuators B: Chemical, Vol.251, pp. 242-249, 2017. https://doi.org/10.1016/j.snb.2017.04.033
- [24] J. Ducrée, S. Haeberle, S. Lutz, S. Pausch, F. Stetten, and R. Zengerle, “The centrifugal microfluidic Bio-Disk platform,” J. of Micromechanics and Microengineering, Vol.17, No.7, pp. 103-115, 2007. https://doi.org/10.1088/0960-1317/17/7/S07
- [25] C. Sobecki, J. Zhang, and C. Wang, “Numerical study of paramagnetic elliptical microparticles in curved channels and uniform magnetic fields,” Micromachines, Vol.11, No.1, Article No.37, 2019. https://doi.org/10.3390/mi11010037
- [26] J. M. Martel, K. C. Smith, M. Dlamini, K. Pletcher, J. Yang, M. Karabacak, D. A. Haber, R. Kapur, and M. Toner, “Continuous flow microfluidic bioparticle concentrator,” Scientific Reports, Vol.5, Article No.11300, 2015. https://doi.org/10.1038/srep11300
- [27] T. S. Sheu, S. J. Chen, and J. Chen, “Mixing of a split and recombine micromixer with tapered curved microchannels,” Chemical engineering science, Vol.71, pp. 321-332, 2012. https://doi.org/10.1016/j.ces.2011.12.042
- [28] A. Shiriny and M. Bayareh, “Inertial focusing of CTCs in a novel spiral microchannel,” Chemical Engineering Science, Vol.229, Article No.116102, 2020. https://doi.org/10.1016/j.ces.2020.116102
- [29] S. S. Kuntaegowdanahalli, A. A. S. Bhagat, G. Kumarb, and I. Papautsky, “Inertial microfluidics for continuous particle separation in spiral microchannels,” Lab on a Chip, Vol.9, No.20, pp. 2973-2980, 2009. https://doi.org/10.1039/B908271A
- [30] G. Guan, L. Wu, A. A. Bhagat, Z. Li, P. C. Y. Chen, S. Chao, C. J. Ong, and J. Han, “Spiral microchannel with rectangular and trapezoidal cross-sections for size based particle separation,” Scientific Reports, Vol.3, Article No.1475, 2013. https://doi.org/10.1038/srep01475
- [31] A. A. S. Bhagat, S. S. Kuntaegowdanahallia, and I. Papautsky, “Continuous particle separation in spiral microchannels using dean flows and differential migration,” Lab on a Chip, Vol.8, No.11, pp. 1906-1914, 2008. https://doi.org/10.1039/B807107A
- [32] P. Y. Yeh, Z. Dai, X. Yang, M. Bergeron, Z. Zhang, M. Lin, and X. Cao, “An efficient spiral microchannel for continuous small particle separations,” Sensors and Actuators B: Chemical, Vol.252, pp. 606-615, 2017. https://doi.org/10.1016/j.snb.2017.06.037
- [33] M. Rafeie, J. Zhang, M. Asadnia, W. Li, and M. E. Warkiani, “Multiplexing slanted spiral microchannels for ultra-fast blood plasma separation,” Lab on a Chip, Vol.16, No.15, pp. 2791-2802, 2016. https://doi.org/10.1039/C6LC00713A
- [34] S. Ghadami, R. Kowsari-Esfahan, M. S. Saidi, and K. Firoozbakhsh, “Spiral microchannel with stair-like cross section for size-based particle separation,” Microfluidics and Nanofluidics, Vol.21, Article No.115, 2017. https://doi.org/10.1007/s10404-017-1950-3
- [35] D. D. Carlo, “Inertial microfluidic,” Lab on a Chip, Vol.9, No.21, pp. 3038-3046, 2009. https://doi.org/10.1039/B912547G
- [36] N. Nivedita, P. Ligrani, and I. Papautsky, “Dean Flow Dynamics in Low-Aspect Ratio Spiral Microchannels,” Scientific Reports, Vol.7, No.1, Article No.44072, 2017. https://doi.org/10.1038/srep44072
- [37] T. Akai, H. Ito, and M. Kaneko, “Deep learning assisted analysis of multiple individual red blood cells in blood flow,” The 22nd Int. Conf. on Miniaturized Systems for Chemistry and Life Sciences (MicroTAS2018), pp. 197-198, 2018.
- [38] R. Otomo and R. Kira, “The Effect of the Layered Internal Structure of Fibrous Beds on the Hydrodynamic Diffusive Behavior of Microparticles,” Micromachines, Vol.12, No.10, Article No.1241, 2021. https://doi.org/10.3390/mi12101241
- [39] S. Shen, F. Zhang, S. Wang, J. Wang, D. Long, D. Wang, and Y. Niu, “Ultra-low aspect ratio spiral microchannel with ordered micro-bars for flow-rate insensitive blood plasma extraction,” Sensors and Actuators B: Chemical, Vol.287, pp. 320-328, 2019. https://doi.org/10.1016/j.snb.2019.02.066
- [40] J. Takagi, M. Yamada, M. Yasuda, and M. Seki, “Continuous particle separation in a microchannel having asymmetrically arranged multiple branches,” Lab on a Chip, Vol.5, No.7, pp. 778-784, 2005. https://doi.org/10.1039/B501885D
- [41] D. H. Yoon, J. Ito, T. Sekiguchi, and S. Shoji, “Active and precise control of microdroplet division using horizontal pneumatic valves in bifurcating microchannel,” Micromachines, Vol.4, No.2, pp. 197-205, 2013. https://doi.org/10.3390/mi4020197
This article is published under a Creative Commons Attribution-NoDerivatives 4.0 Internationa License.