Review:
Motor Characteristics of Human Adaptations to External Assistive Forces
Wen Liang Yeoh*
, Jeewon Choi**
, Ping Yeap Loh***
, Osamu Fukuda*
, and Satoshi Muraki***

*Faculty of Science and Engineering, Saga University
1 Honjo-machi, Saga 840-8502, Japan
**Department of Industrial and Management Systems Engineering, Dong-A University
37 Nakdong-daero, 550 Beon-gil, Saha-gu, Busan 49315, Korea
***Faculty of Design, Kyushu University
4-9-1 Shiobaru, Minami-ku, Fukuoka 815-8540, Japan
Technology advancement has enabled the development of robotic exoskeletons that are portable, powerful, and sufficiently smart to be of practical use in the real world. These devices provide partial assistive forces that increase their user’s physical strength to better meet the demands of everyday life and have potential applications in various settings. Examples include helping older adults maintain their independence and preventing musculoskeletal injuries among factory workers. Although great strides have been made to improve the performance and usability of these devices, human characteristics and the way humans adapt to the external assistive forces from these devices are rarely explicitly considered in their development. A common assumption is that if the provided assistive forces are aligned with the intent of users, users can easily “switch off” their muscles and effectively utilize this assistive force. In this review, we demonstrate that human adaptations to external assistive forces can lead to inefficiencies or conflicts that decrease the effectiveness of robotic exoskeletons. We then discuss the motor characteristics of human adaptations to external assistive forces.
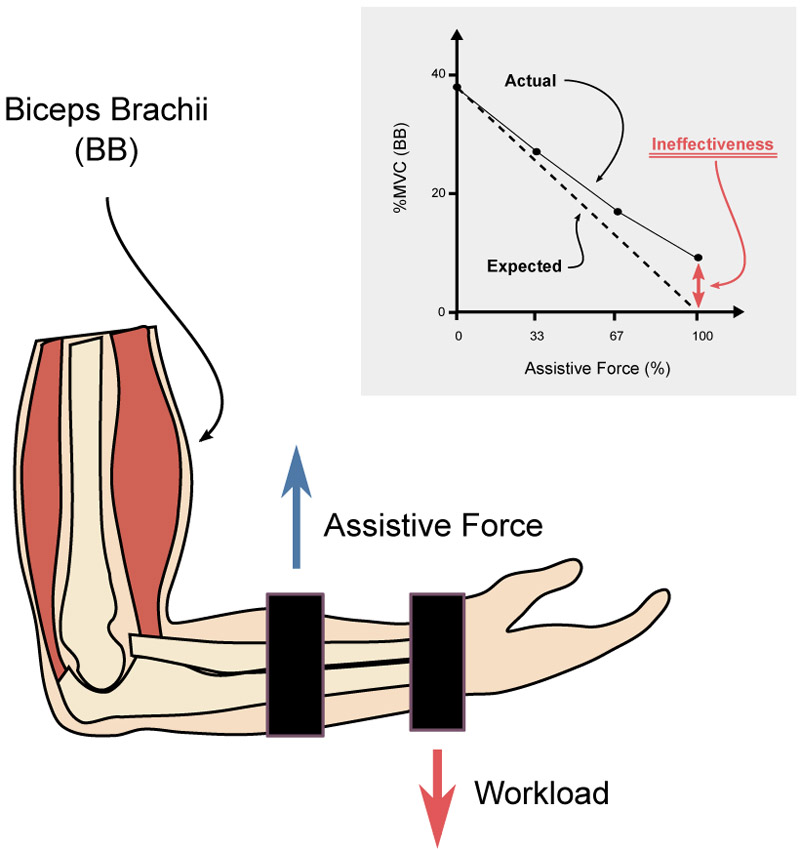
Ineffectiveness of assistive forces
- [1] G. Bao et al., “Academic Review and Perspectives on Robotic Exoskeletons,” IEEE Trans. Neural Syst. Rehabil. Eng., Vol.27, No.11, pp. 2294-2304, Nov. 2019. https://doi.org/10.1109/TNSRE.2019.2944655
- [2] A. Ebrahimi, D. Gröninger, R. Singer, and U. Schneider, “Control parameter optimization of the actively powered upper body exoskeleton using subjective feedbacks,” 2017 3rd Int. Conf. on Control, Automation and Robotics (ICCAR), pp. 432-437, Apr. 2017. https://doi.org/10.1109/ICCAR.2017.7942733
- [3] R. A. R. C. Gopura, D. S. V. Bandara, K. Kiguchi, and G. K. I. Mann, “Developments in hardware systems of active upper-limb exoskeleton robots: A review,” Robot. Auton. Syst., Vol.75, Part B, pp. 203-220, Jan. 2016. https://doi.org/10.1016/j.robot.2015.10.001
- [4] A. Nilsson, K. S. Vreede, V. Häglund, H. Kawamoto, Y. Sankai, and J. Borg, “Gait training early after stroke with a new exoskeleton – the hybrid assistive limb: A study of safety and feasibility,” J. NeuroEngineering Rehabil., Vol.11, No.1, Article No.92, Jun. 2014. https://doi.org/10.1186/1743-0003-11-92
- [5] J. Beil, G. Perner, and T. Asfour, “Design and control of the lower limb exoskeleton KIT-EXO-1,” 2015 IEEE Int. Conf. on Rehabilitation Robotics (ICORR), pp. 119-124, Aug. 2015. https://doi.org/10.1109/ICORR.2015.7281186
- [6] C. Thakur, K. Ogawa, and Y. Kurita, “Active Passive Nature of Assistive Wearable Gait Augment Suit for Enhanced Mobility,” J. Robot. Mechatron., Vol.30, No.5, pp. 717-728, Oct. 2018. https://doi.org/10.20965/jrm.2018.p0717
- [7] M. Kashima et al., “Development of Assist Suit for Squat Lifting Support Considering Gait and Quantitative Evaluation by Three-Dimensional Motion Analysis,” J. Robot. Mechatron., Vol.32, No.1, pp. 209-219, Feb. 2020. https://doi.org/10.20965/jrm.2020.p0209
- [8] T. Kosaki and S. Li, “A Water-Hydraulic Upper-Limb Assistive Exoskeleton System with Displacement Estimation,” J. Robot. Mechatron., Vol.32, No.1, pp. 149-156, Feb. 2020. https://doi.org/10.20965/jrm.2020.p0149
- [9] H. Lee, B. Lee, W. Kim, M. Gil, J. Han, and C. Han, “Human-robot cooperative control based on pHRI (Physical Human-Robot Interaction) of exoskeleton robot for a human upper extremity,” Int. J. Precis. Eng. Manuf., Vol.13, No.6, pp. 985-992, May 2012. https://doi.org/10.1007/s12541-012-0128-x
- [10] B. H. Goodpaster et al., “The loss of skeletal muscle strength, mass, and quality in older adults: The health, aging and body composition study,” J. Gerontol. A. Biol. Sci. Med. Sci., Vol.61, No.10, pp. 1059-1064, Oct. 2006. https://doi.org/10.1093/gerona/61.10.1059
- [11] D. X. M. Wang, J. Yao, Y. Zirek, E. M. Reijnierse, and A. B. Maier, “Muscle mass, strength, and physical performance predicting activities of daily living: A meta-analysis,” J. Cachexia Sarcopenia Muscle, Vol.11, No.1, pp. 3-25, 2020. https://doi.org/10.1002/jcsm.12502
- [12] G. S. Sawicki, O. N. Beck, I. Kang, and A. J. Young, “The exoskeleton expansion: improving walking and running economy,” J. NeuroEngineering Rehabil., Vol.17, Article No.25, Feb. 2020. https://doi.org/10.1186/s12984-020-00663-9
- [13] S. Toxiri et al., “Rationale, Implementation and Evaluation of Assistive Strategies for an Active Back-Support Exoskeleton,” Front. Robot. AI, Vol.5, 2018. https://doi.org/10.3389/frobt.2018.00053
- [14] Y.-X. Liu, L. Zhang, R. Wang, C. Smith, and E. M. Gutierrez-Farewik, “Weight Distribution of a Knee Exoskeleton Influences Muscle Activities During Movements,” IEEE Access, Vol.9, pp. 91614-91624, 2021. https://doi.org/10.1109/ACCESS.2021.3091649
- [15] I. Priadythama, W. L. Yeoh, P. Y. Loh, and S. Muraki, “The Effect of the Degree of Freedom and Weight of the Hand Exoskeleton on Joint Mobility Function,” Robotics, Vol.11, No.2, Article No.53, Apr. 2022. https://doi.org/10.3390/robotics11020053
- [16] X. Jin, Y. Cai, A. Prado, and S. K. Agrawal, “Effects of exoskeleton weight and inertia on human walking,” 2017 IEEE Int. Conf. on Robotics and Automation (ICRA), pp. 1772-1777, May 2017. https://doi.org/10.1109/ICRA.2017.7989210
- [17] M. B. Näf, K. Junius, M. Rossini, C. Rodriguez-Guerrero, B. Vanderborght, and D. Lefeber, “Misalignment Compensation for Full Human-Exoskeleton Kinematic Compatibility: State of the Art and Evaluation,” Appl. Mech. Rev., Vol.70, No.5, Feb. 2019. https://doi.org/10.1115/1.4042523
- [18] D. Verdel, G. Sahm, S. Bastide, O. Bruneau, B. Berret, and N. Vignais, “Influence of the Physical Interface on the Quality of Human–Exoskeleton Interaction,” IEEE Trans. Hum.-Mach. Syst., Vol.53, No.1, pp. 44-53, 2022. https://doi.org/10.1109/THMS.2022.3175415
- [19] Ž. Kozinc, J. Babič, and N. Šarabon, “Human pressure tolerance and effects of different padding materials with implications for development of exoskeletons and similar devices,” Appl. Ergon., Vol.93, Article No.103379, May 2021. https://doi.org/10.1016/j.apergo.2021.103379
- [20] M. B. Yandell, B. T. Quinlivan, D. Popov, C. Walsh, and K. E. Zelik, “Physical interface dynamics alter how robotic exosuits augment human movement: implications for optimizing wearable assistive devices,” J. NeuroEngineering Rehabil., Vol.14, 2017. https://doi.org/10.1186/s12984-017-0247-9
- [21] A. J. Young and D. P. Ferris, “State of the Art and Future Directions for Lower Limb Robotic Exoskeletons,” IEEE Trans. Neural Syst. Rehabil. Eng., Vol.25, No.2, pp. 171-182, Feb. 2017. https://doi.org/10.1109/TNSRE.2016.2521160
- [22] M. R. Tucker et al., “Control strategies for active lower extremity prosthetics and orthotics: A review,” J. NeuroEngineering Rehabil., Vol.12, Article No.1, Jan. 2015. https://doi.org/10.1186/1743-0003-12-1
- [23] R. Baud, A. R. Manzoori, A. Ijspeert, and M. Bouri, “Review of control strategies for lower-limb exoskeletons to assist gait,” J. NeuroEngineering Rehabil., Vol.18, Article No.119, Jul. 2021. https://doi.org/10.1186/s12984-021-00906-3
- [24] Y. Li et al., “A review on interaction control for contact robots through intent detection,” Prog. Biomed. Eng., Vol.4, No.3, Article No.032004, Jul. 2022. https://doi.org/10.1088/2516-1091/ac8193
- [25] B. Chen, C.-H. Zhong, X. Zhao, H. Ma, L. Qin, and W.-H. Liao, “Reference Joint Trajectories Generation of CUHK-EXO Exoskeleton for System Balance in Walking Assistance,” IEEE Access, Vol.7, pp. 33809-33821, 2019. https://doi.org/10.1109/ACCESS.2019.2904296
- [26] G. S. Sawicki, A. Domingo, and D. P. Ferris, “The effects of powered ankle-foot orthoses on joint kinematics and muscle activation during walking in individuals with incomplete spinal cord injury,” J. NeuroEngineering Rehabil., Vol.3, Article No.3, Feb. 2006. https://doi.org/10.1186/1743-0003-3-3
- [27] Y. Guo, W. Xu, S. Pradhan, C. Bravo, and P. Ben-Tzvi, “Personalized voice activated grasping system for a robotic exoskeleton glove,” Mechatronics, Vol.83, Article No.102745, May 2022. https://doi.org/10.1016/j.mechatronics.2022.102745
- [28] T.-H. Hsu, Y.-C. Chiang, W.-T. Chan, and S.-J. Chen, “A Finger Exoskeleton Robot for Finger Movement Rehabilitation,” Inventions, Vol.2, No.3, Jul. 2017. https://doi.org/10.3390/inventions2030012
- [29] Y. He, R. Kubozono, O. Fukuda, N. Yamaguchi, and H. Okumura, “Vision-Based Assistance for Myoelectric Hand Control,” IEEE Access, Vol.8, pp. 201956-201965, 2020. https://doi.org/10.1109/ACCESS.2020.3036115
- [30] S. Veselic, C. Zito, and D. Farina, “Human-Robot Interaction with Robust Prediction of Movement Intention Surpasses Manual Control,” Front. Neurorobotics, Vol.15, 2021. https://doi.org/10.3389/fnbot.2021.695022
- [31] D. P. Losey, C. G. McDonald, E. Battaglia, and M. K. O’Malley, “A Review of Intent Detection, Arbitration, and Communication Aspects of Shared Control for Physical Human–Robot Interaction,” Appl. Mech. Rev., Vol.70, No.1, Article No.010804, Jan. 2018. https://doi.org/10.1115/1.4039145
- [32] K. Anam and A. A. Al-Jumaily, “Active Exoskeleton Control Systems: State of the Art,” Procedia Eng., Vol.41, pp. 988-994, Jan. 2012. https://doi.org/10.1016/j.proeng.2012.07.273
- [33] M. A. Gull, S. Bai, and T. Bak, “A Review on Design of Upper Limb Exoskeletons,” Robotics, Vol.9, No.1, Mar. 2020. https://doi.org/10.3390/robotics9010016
- [34] N. Hogan and S. P. Buerger, “Impedance and interaction control,” Robotics and Automation Handbook, CRC Press, pp. 375-398, 2018.
- [35] A. R. Wu et al., “An Adaptive Neuromuscular Controller for Assistive Lower-Limb Exoskeletons: A Preliminary Study on Subjects with Spinal Cord Injury,” Front. Neurorobotics, Vol.11, 2017. https://doi.org/10.3389/fnbot.2017.00030
- [36] J. M. Winters, “Hill-based muscle models: a systems engineering perspective,” Multiple Muscle Systems, Springer, pp. 69-93, 1990. https://doi.org/10.1007/978-1-4613-9030-5_5
- [37] H. Geyer and H. Herr, “A Muscle-Reflex Model That Encodes Principles of Legged Mechanics Produces Human Walking Dynamics and Muscle Activities,” IEEE Trans. Neural Syst. Rehabil. Eng., Vol.18, No.3, pp. 263-273, Jun. 2010. https://doi.org/10.1109/TNSRE.2010.2047592
- [38] N. Nasir, K. Hayashi, P. Y. Loh, and S. Muraki, “The Effect of Assistive Force on The Agonist and Antagonist Muscles in Elbow Flexion,” Mov. Health Exerc., Vol.6, No.2, p. 35, Jan. 2017. https://doi.org/10.15282/mohe.v6i2.139
- [39] P. Y. Loh, K. Hayashi, N. Nasir, and S. Muraki, “Changes in Muscle Activity in Response to Assistive Force During Isometric Elbow Flexion,” J. Mot. Behav., Vol.52, No.5, pp. 634-642, Sep. 2020. https://doi.org/10.1080/00222895.2019.1670128
- [40] M. W. R. Holmes and P. J. Keir, “Posture and hand load alter muscular response to sudden elbow perturbations,” J. Electromyogr. Kinesiol., Vol.22, No.2, pp. 191-198, Apr. 2012. https://doi.org/10.1016/j.jelekin.2011.11.006
- [41] T. E. Milner, “Contribution of geometry and joint stiffness to mechanical stability of the human arm,” Exp. Brain Res., Vol.143, No.4, pp. 515-519, Apr. 2002. https://doi.org/10.1007/s00221-002-1049-1
- [42] U. Proske and S. C. Gandevia, “The Proprioceptive Senses: Their Roles in Signaling Body Shape, Body Position and Movement, and Muscle Force,” Physiol. Rev., Vol.92, No.4, pp. 1651-1697, Oct. 2012. https://doi.org/10.1152/physrev.00048.2011
- [43] M. B. Spraker, D. M. Corcos, and D. E. Vaillancourt, “Cortical and Subcortical Mechanisms for Precisely Controlled Force Generation and Force Relaxation,” Cereb. Cortex, Vol.19, No.11, pp. 2640-2650, Nov. 2009. https://doi.org/10.1093/cercor/bhp015
- [44] K. B. Harbst, J.-A. C. Lazarus, and J. Whitall, “Accuracy of Dynamic Isometric Force Production: The Influence of Age and Bimanual Activation Patterns,” Motor Control, Vol.4, No.2, pp. 232-256, Apr. 2000. https://doi.org/10.1123/mcj.4.2.232
- [45] J. Masumoto and N. Inui, “Control of increasing or decreasing force during periodic isometric movement of the finger,” Hum. Mov. Sci., Vol.29, No.3, pp. 339-348, Jun. 2010. https://doi.org/10.1016/j.humov.2009.11.006
- [46] K. Kato, T. Muraoka, T. Higuchi, N. Mizuguchi, and K. Kanosue, “Interaction between simultaneous contraction and relaxation in different limbs,” Exp. Brain Res., Vol.232, No.1, pp. 181-189, Jan. 2014. https://doi.org/10.1007/s00221-013-3730-y
- [47] S. Sakurai and T. Ohtsuki, “Muscle activity and accuracy of performance of the smash stroke in badminton with reference to skill and practice,” J. Sports Sci., Vol.18, No.11, pp. 901-914, Jan. 2000. https://doi.org/10.1080/026404100750017832
- [48] J. Choi, W. L. Yeoh, P. Y. Loh, and S. Muraki, “Force and electromyography responses during isometric force release of different rates and step-down magnitudes,” Hum. Mov. Sci., Vol.67, Article No.102516, Oct. 2019. https://doi.org/10.1016/j.humov.2019.102516
- [49] C. Ohtaka and M. Fujiwara, “Control Strategies for Accurate Force Generation and Relaxation,” Percept. Mot. Skills, Vol.123, No.2, pp. 489-507, Oct. 2016. https://doi.org/10.1177/0031512516664778
- [50] J. Choi, W. L. Yeoh, S. Matsuura, P. Y. Loh, and S. Muraki, “Effects of mechanical assistance on muscle activity and motor performance during isometric elbow flexion,” J. Electromyogr. Kinesiol., Vol.50, Article No.102380, Feb. 2020. https://doi.org/10.1016/j.jelekin.2019.102380
- [51] A. Adam, C. J. D. Luca, and Z. Erim, “Hand Dominance and Motor Unit Firing Behavior,” J. Neurophysiol., Vol.80, No.3, pp. 1373-1382, Sep. 1998. https://doi.org/10.1152/jn.1998.80.3.1373
- [52] R. L. Sainburg and D. Kalakanis, “Differences in Control of Limb Dynamics During Dominant and Nondominant Arm Reaching,” J. Neurophysiol., Vol.83, No.5, pp. 2661-2675, May 2000. https://doi.org/10.1152/jn.2000.83.5.2661
- [53] Y. Wang, J. Choi, P. Y. Loh, and S. Muraki, “A comparison of motor control characteristics of the dominant and non-dominant arms in response to assistive force during unilateral task,” Isokinet. Exerc. Sci., Vol.27, No.4, pp. 313-324, Jan. 2019. https://doi.org/10.3233/IES-193165
- [54] C. N. Schabowsky, J. M. Hidler, and P. S. Lum, “Greater reliance on impedance control in the nondominant arm compared with the dominant arm when adapting to a novel dynamic environment,” Exp. Brain Res., Vol.182, No.4, pp. 567-577, Oct. 2007. https://doi.org/10.1007/s00221-007-1017-x
- [55] H. Kousaka, H. Mizoguchi, M. Yoshikawa, H. Tanaka, and Y. Matsumoto, “Role Analysis of Dominant and Non-Dominant Hand in Daily Life,” 2013 IEEE Int. Conf. on Systems, Man, and Cybernetics, pp. 3972-3977, Oct. 2013. https://doi.org/10.1109/SMC.2013.678
- [56] K. Kato, T. Vogt, and K. Kanosue, “Brain Activity Underlying Muscle Relaxation,” Front. Physiol., Vol.10, 2019. https://doi.org/10.3389/fphys.2019.01457
- [57] J. Choi, W. L. Yeoh, P. Y. Loh, and S. Muraki, “Motor performance patterns between unilateral mechanical assistance and bilateral muscle contraction,” Int. J. Ind. Ergon., Vol.80, Article No.103056, Nov. 2020. https://doi.org/10.1016/j.ergon.2020.103056
- [58] Y. Wang, P. Y. Loh, and S. Muraki, “Motor control characteristics of upper limbs in response to assistive forces during bilateral tasks,” PLoS ONE, Vol.16, No.1, Article No.e0245049, 2021. https://doi.org/10.1371/journal.pone.0245049
- [59] D. E. Rassier, B. R. MacIntosh, and W. Herzog, “Length dependence of active force production in skeletal muscle,” J. Appl. Physiol., Vol.86, No.5, pp. 1445-1457, 1999. https://doi.org/10.1152/jappl.1999.86.5.1445
- [60] R. W. Gülch, “Force-velocity relations in human skeletal muscle,” Int. J. Sports Med., Vol.15, pp. S2-S10, 1994. https://doi.org/10.1055/s-2007-1021103
- [61] C. Ohtaka and M. Fujiwara, “Force control characteristics for generation and relaxation compared between the upper and lower limbs,” J. Hum. Sport Exerc., Vol.17, No.1, pp. 181-196, Jan. 2022. https://doi.org/10.14198/jhse.2022.171.17
- [62] S. Muraki, J. Choi, S. Saito, W. L. Yeoh, P. Y. Loh, and H. Flores, “Assistive technology considering human characteristics. Design guideline for power assistance,” Kyushu University, Oct. 2022. https://doi.org/10.15017/4843905
This article is published under a Creative Commons Attribution-NoDerivatives 4.0 Internationa License.