Paper:
Development of the MRI Flow Phantom System Focused on Low Speed Flows in Fluid Machinery
Kazunori Hosotani*1, Shota Uehara*1, Toru Ishihara*1, Atsushi Ono*2, Kazuhiro Takeuchi*3, and Yusuke Hashiguchi*4
*1National Institute of Technology, Tsuyama College
624-1 Numa, Tsuyama-shi, Okayama 708-8509, Japan
*2Kawasaki University of Medical Welfare
288 Matsushima, Kurashiki-shi, Okayama 701-0193, Japan
*3National Hospital Organization, Okayama Medical Center
1711-1 Tamasu, Kita-ku, Okayama-shi, Okayama 701-1192, Japan
*4Kousei Hospital
3-8-35 Kousei-cho, Kita-ku, Okayama-shi, Okayama 700-0985, Japan
At present, magnetic resonance imaging (MRI), which is one of the noninvasive diagnostic imaging techniques for medical use, has been applied to industrial product inspection. In this study, a hydraulic model test system, called the “flow phantom system,” which can generate steady flow and oscillating flow for time-resolved MRI, was developed to aid optimum design of fluid machinery. Simple flow phantoms are tested under laminar flow or oscillating flow to evaluate the validity and effectiveness of the proposed system. In this article, the test results of double cylindrical pipe flow, elbow pipe flow, cylindrical valve flow, and jet pump flow, which are often seen in fluid machines, are tested using the 2D time-spatial labeling inversion pulse (Time-SLIP) method, which can track a labeled water mass and visualize it using two-dimensional images. MRI-detected flow patterns were compared with particle image velocimetry (PIV) or numerical simulation.
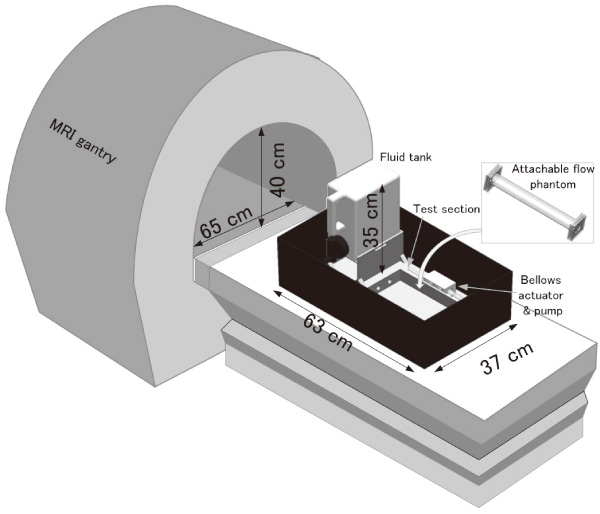
Conceptual diagram of the flow phantom system
- [1] G. Matsui and H. Monji, “Full-field visualization measurement in fluid mechanics,” J. the Japanese Society for Experimental Mechanics, Vol.2, No.1, pp. 3-8, 2002.
- [2] S. Wada, K. Tezuka, W. Treenuson, N. Tsuzuki, and H. Kikura, “Study on the optimal number of transducers for pipe flow rate measurement downstream of a single elbow using the Ultrasonic Velocity Profile method,” Science and Technology of Nuclear Installations, Vol.2012, pp. 1-12.
- [3] I. V. Koptyug, S. I. Kabanikhin, K. T. Iskakov, V. B. Fenelonov, L. Y. Khitrina, R. Z. Sagdeev, and V. N. Parmon, “A quantitative NMR imaging study of mass transport in porous solids during drying,” Chemical Engineering Science, Vol.55, pp. 1559-1571, 2000.
- [4] I. V. Koptyug, L. Y. Ilyina, A. V. Matveev, R. Z. Sagdeev, V. N. Parmon, and S. A. Altobelli, “Liquid and gas flow and related phenomena in monolithic catalysts studied by 1H NMR microimaging,” Catalysis Today, Vol.69, pp. 385-392, 2001.
- [5] C. Hilty, E. McDonnell, J. Granwehr, K. Pierce, S. Han, and A. Pines, “Microfuidic gas-fow profiling using remote-detection NMR,” Proc. the National Academy of Sciences of the United States of America, Vol.102, No.42, pp. 14960-14963, 2005.
- [6] S. Tsushima and S. Hirai, “Magnetic resonance imaging of water in operating polymer electrolyte membrane fuel cells,” Fuel Cells, Vol.9, No.5, pp. 506-517, 2009.
- [7] M. Markl, F. P. Chan, M. T. Alley, K. L. Wedding, M. T. Draney, C. J. Elkins, D. W. Parker, R. Wicker, C. A. Taylor, R. J. Herfkens, and N. J. Pelc, “Time-resolved three-dimensional phase-contrast MRI,” J. Magn. Reson. Imaging, Vol.17, pp. 499-506, 2003.
- [8] Y. Wang, S.-E. Kim, E. Dibella, and D. L. Parker, “Flow measurement in MRI using arterial spin labeling with cumulative readout pulses – Theory and validation,” J. Medical Physics, Vol.37, No.11, pp. 5801-5810, 2010.
- [9] K. Takeuchi, A. Ono, Y. Hashiguchi, H. Misawa, T. Takahata, A. Teramoto, and S. Nakahara, “Visualization of cerebrospinal fluid flow in syringomyelia through noninvasive magnetic resonance imaging with a time-spatial labeling inversion pulse (Time-SLIP),” J. Spinal Cord Med., Vol.40, No.3, pp. 368-371, 2017.
- [10] H. Isoda, M. Hirano, H. Takeda, T. Kosugi, M. T. Alley, M. Markl, N. J. Pelc, and H. Sakahara, “Visualization of hemodynamics in a silicon aneurysm model using time-resolved 3D phase-contrast MRI,” AJNR Am J. Neuroradiol, Vol.27, pp. 1119-1122, 2006.
- [11] F. Ong, M. Uecker, U. Tariq, A. Hsiao, M. T. Alley, S. S. Vasanawala, and M. Lustig, “Robust 4D flow denoising using divergence-free wavelet transform,” Magn Reson Med., Vol.73, No.2, pp. 828-842, 2015.
- [12] S. Thyagaraj, S. H. Pahlavian, L. R. Sass, F. Loth, M. Vatani, J. W. Choi, R. S. Tubbs, D. Giese, J. R. Kroger, A. C. Bunck, and B. A. Martin, “An MRI-compatible hydrodynamic simulator of cerebrospinal fluid motion in the cervical spine,” IEEE Trans. Biomed Eng., Vol.26, doi: 10.1109/TBME.2017.2756995, 2017.
- [13] S. H. Pahlavian, A. C. Bunck, F. Loth, R. S. Tubbs, T. Yiallourou, J. R. Kroeger, W. Heindel, and B. A. Martin, “Characterization of the discrepancies between four-dimensional phase-contrast magnetic resonance imaging and in-silico simulations of cerebrospinal fluid dynamics,” J. Biomech Eng., Vol.137, No.5, 051002, 2015.
- [14] H. Ha, J. Lantz, H. Haraldsson, B. Casas, M. Ziegler, M. Karlsson, D. Saloner, P. Dyverfeldt, and T. Ebbers, “Assessment of turbulent viscous stress using ICOSA 4D Flow MRI for prediction of hemodynamic blood damage,” Scientific Reports, Vol.6, 39773, 2016.
- [15] K. Hosotani, A. Ono, K. Takeuchi, Y. Hashiguchi, and T. Nagahata, “Flow Visualization of simple pipe and channel flows obtained by the MRI time-slip method,” J. Visualization, doi: 10.1007/s12650-016-0395-1, 2016.
- [16] M. Paley, R. Hose, I. Marzouqa, J. Fenner, I. Wilkinson, Y. Noguchi, and P. Griffiths, “Stable periodic vortex shedding studied using computational fluid dynamics, laser sheet flow visualization, and MR imaging,” Magnetic Resonance Imaging, Vol.18, No.4, pp. 473-479, 2000.
- [17] I. Ashino, “Theory on laminar flow between eccentric straight pipes,” Trans. of the Japan Society of Mechanical Engineers, Vol.31, No.223, pp. 407-4115, 1965.
- [18] T. Kawaguchi, “MRI measurement of granular flows and fluid-particle flows,” Advanced Powder Technology, Vol.21, No.3, pp. 235-241, 2010.
This article is published under a Creative Commons Attribution-NoDerivatives 4.0 Internationa License.