Paper:
Visual Lifting Approach for Bipedal Walking with Slippage
Xiang Li, Mamoru Minami, Takayuki Matsuno, and Daiji Izawa
Graduate School of Nature Science and Technology, Okayama University
3-1-1 Tsushima-naka, Kita-ku, Okayama-shi, Okayama 700-8530, Japan
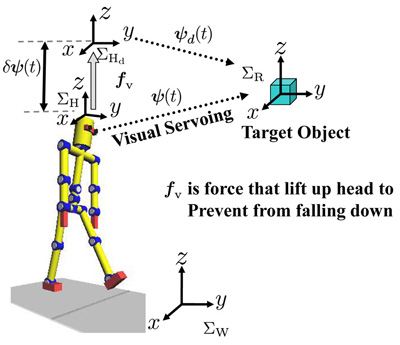
Concept of visual lifting approach
- [1] M. Vukobratovic, A. Frank, and D. Juricic, “On the Stability of Biped Locomotion,” IEEE Trans. on Biomedical Engineering, Vol.17, No.1, 1970.
- [2] M. Vukobratovic and J. Stepanenko, “On the Stability of Anthropomorphic Systems,” Mathematical Biosciences, Vol.15, pp. 1-37, 1972.
- [3] S. Colins, A. Ruina, R. Tedrake, and M. Wisse, “Efficient Bipedal Robots Based on Passive-Dynamic Walkers,” Science, Vol.307, pp. 1082-1085, 2005.
- [4] J. Pratt, P. Dilworth, and G. Pratt, “Virtual Model Control of a Bipedal Walking Robot,” Proc. of IEEE Int. Conf. on Robotics and Automation,” pp. 193-198, 1997.
- [5] R. E. Westervelt, W. J. Grizzle, and E. D. Koditschek, “Hybrid Zero Dynamics of Planar Biped Walkers,” IEEE Trans. on Automatic Control, Vol.48, No.1, pp. 42-56, 2003.
- [6] Y. Harada, J. Takahashi, D. Nenchev, and D. Sato, “Limit Cycle Based Walk of a Powered 7DOF 3D Biped with Flat Feet,” Proc. of IEEE/RSJ Int. Conf. on Intelligent Robots and Systems, pp. 3623-3628, 2010.
- [7] Y. Huang, B. Chen, Q. Wang, K. Wei, and L. Wang, “Energetic efficiency and stability of dynamic bipedal walking gaits with different step lengths,” Proc. of IEEE/RSJ Int. Conf. on Intelligent Robots and Systems, pp. 4077-4082, 2010.
- [8] D. Peng and K. G. Shin, “Modeling of Concurrent Task Execution in a Distributed System for Real-Time Control,” IEEE Trans. on Computers, Vol.C-36, No.4, pp. 510-516, April 1987.
- [9] T. Wu, T. Yeh, and B. Hsu, “Trajectory Planning of a One-Legged Robot Performing Stable Hop,” Proc. of IEEE/RSJ Int. Conf. on Intelligent Robots and Systems, pp. 4922-4927, 2010.
- [10] Y. Nakamura and K. Yamane, “Dynamics of Kinematic Chains with Discontinuous Changes of Constraints – Application to Human Figures that Move in Contact with the Environments –,” J. of RSJ, Vol.18, No.3, pp. 435-443, 2000 (in Japanese).
- [11] K. Yamane and Y. Nakamura, “Dynamics Filter – Concept and Implementation of On-Line Motion Generator for Human Figures,” IEEE Trans. on Robotics and Automation, Vol.19, No.3, pp. 421-432, 2003.
- [12] X. Li, H. Imanishi, M. Minami, T. Matsuno, and A. Yanou, “Dynamical Model of Walking Transition Considering Nonlinear Friction with Floor,” J. of Advanced Computational Intelligence and Intelligent Informatics, Vol.20, No.6, 2016.
- [13] W. Song, M. Minami, and Y. Zhang, “A Visual Lifting Approach for Dynamic Bipedal Walking,” Int. J. of Advanced Robotic Systems, Vol.9, pp. 1-8, 2012.
- [14] A. Yanou, M. Minami, T. Maeba, and Y. Kobayashi, “A First Step of Humanoid’s Walking by Two Degree-of-freedom Generalized Predictive Control Combined with Visual Lifting Stabilization,” Proc. of the 39th Annual Conf. of the IEEE Industrial Electronics Society (IECON2013), pp. 6357-6362, 2013.
- [15] W. Song, M. Minami, T. Maeba, Y. Zhang, and A. Yanou, “Visual Lifting Stabilization of Dynamic Bipedal Walking,” Proc. of 2011 IEEE-RAS Int. Conf. on Humanoid Robots, pp. 345-351, 2011.
- [16] N. Hogan, “Impedance Control; An Approach to Manipulation, Parts I–III,” ASME J. of Dynamics Systems, Measurement, and Control Vol.107, No.1, pp. 1-24, 1985.
- [17] W. Song, M. Minami, F. Yu, Y. Zhang, and A. Yanou, “3-D Hand & Eye-Vergence Approaching Visual Servoing with Lyapunov-Stable Pose Tracking,” Proc. of IEEE Int. Conf. on Robotics and Automation, pp. 5210-5217, 2011.
- [18] F. Yu, W. Song, and M. Minami, “Visual Servoing with Quick Eye-Vergence to Enhance Trackability and Stability,” Proc. of IEEE/RSJ Int. Conf. on Intelligent Robots and Systems, pp. 6228-6233, 2010.
- [19] M. Kouchi, M. Mochimaru, H. Iwasawa, and S. Mitani, “Anthropometric database for Japanese Population 1997-98,” Japanese Industrial Standards Center (AIST, MITI), 2000.
- [20] T. Maeba, M. Minami, A. Yanou, and J. Nishiguchi, “Dynamical Analyses of Humanoid’s Walking by Visual Lifting Stabilization Based on Event-driven State Transition,” 2012 IEEE/ASME Int. Conf. on Advanced Intelligent Mechatronics Proc., pp. 7-14, 2012.
This article is published under a Creative Commons Attribution-NoDerivatives 4.0 Internationa License.