Paper:
Single-Use Aircraft Launch System with Small Helium Balloon for 1 kPa Flight Testing
Hikaru Otsuka
, Hiromi Ueno, Renta Oishi, Naoki Hayashi, Ryoma Nakayama, and Hiroshi Tokutake
Kanazawa University
Kakuma-machi, Kanazawa, Ishikawa 920-1192, Japan
A single-use aircraft launch system using a small helium balloon concept was proposed to elevate the likelihood of successful high-altitude flight tests above 30 km. The system, capable of carrying a payload of 0.5 kg, has been developed and reported. Completing the flight within a range of 92 km, which represents the maximum communication distance between the airborne flight system and the ground station for operational phase control, is imperative. Therefore, a straightforward method for simulating flight trajectories was outlined to determine launch windows and suitable locations to ensure flight completion within uninhabited areas. Simulation studies revealed that the shortest landing distance was obtained during summer at the selected launch site. Additionally, a parameter study was conducted to assess the influence of varying ascent velocities on the targeted flight test altitude, which depends on the payload weight and helium gas volume. Consequently, the parameter study results established the constraints for flight model design. A demonstration flight was conducted in 2023 to validate the feasibility of the system for high-altitude flight experiments. This flight successfully released the flight model at 31 km and recovered flight data before landing on the sea surface. The proposed system concept offers an expedited approach to aircraft design for operating in thin air. It enables component function tests and scaled model flights as an alternative to employing large high-altitude balloons for high-altitude experiments.
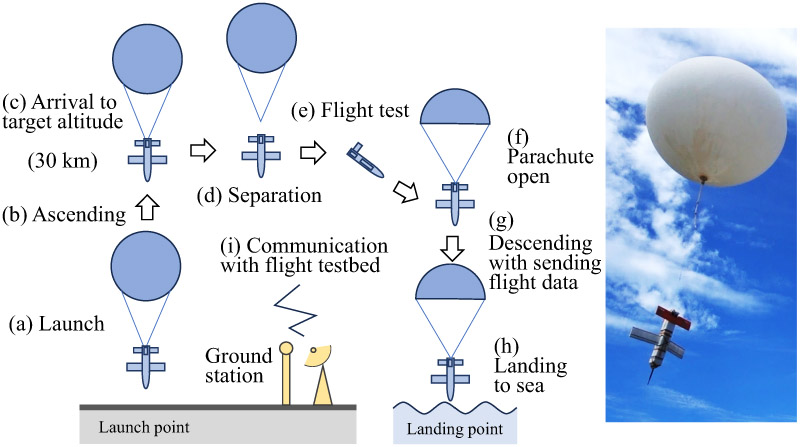
Single-use high-altitude flight system
- [1] K. A. Farley et al., “Mars 2020 mission overview,” Space Sci. Rev., Vol.216, No.8, Article No.142, 2020. https://doi.org/10.1007/s11214-020-00762-y
- [2] M. Jesick et al., “Navigation overview for the Mars atmosphere and volatile evolution mission,” J. Spacecr. Rockets, Vol.54, No.1, pp. 29-43, 2017. https://doi.org/10.2514/1.A33618
- [3] T. Tzanetos et al., “Ingenuity Mars helicopter: From technology demonstration to extraterrestrial scout,” 2022 IEEE Aerosp. Conf., 2022. https://doi.org/10.1109/AERO53065.2022.9843428
- [4] R. D. Braun, H. S. Wright, M. A. Croom, J. S. Levine, and D. A. Spencer, “Design of the ARES Mars airplane and mission architecture,” J. Spacecr. Rockets, Vol.43, No.5, pp. 1026-1034, 2006. https://doi.org/10.2514/1.17956
- [5] K. Fujita, R. Luong, H. Nagai, and K. Asai, “Conceptual design of Mars airplane,” Trans. Jpn. Soc. Aeronaut. Space Sci., Aerosp. Technol. Jpn., Vol.10, No.ists28, pp. Te_5-Te_10, 2012. https://doi.org/10.2322/tastj.10.Te_5
- [6] D. Banfield et al., “The atmosphere of Mars as observed by InSight.” Nat. Geosci., Vol.13, No.3, pp. 190-198, 2020. https://doi.org/10.1038/s41561-020-0534-0
- [7] M. Anyoji et al., “Computational and experimental analysis of a high-performance airfoil under low-Reynolds-number flow condition,” J. Aircr., Vol.51, No.6, pp. 1864-1872, 2014. https://doi.org/10.2514/1.C032553
- [8] H. Tokutake, K. Fujita, A. Oyama, and H. Nagai, “Attitude estimation using thermopile sensors on Mars airplane balloon experiment-1 (MABE-1),” Trans. Jpn. Soc. Aeronaut. Space Sci., Aerosp. Technol. Jpn., Vol.18, No.2, pp. 17-24, 2020. https://doi.org/10.2322/tastj.18.17
- [9] K. Fujita, H. Tokutake, H. Nagai, and A. Oyama, “Flight control parameter design for Mars airplane balloon experiment-1 (MABE-1) using evolutionary computation,” Trans. Jpn. Soc. Aeronaut. Space Sci., Aerosp. Technol. Jpn., Vol.17, No.4, pp. 512-518, 2019. https://doi.org/10.2322/tastj.17.512
- [10] M. Anyoji, M. Okamoto, K. Fujita, H. Nagai, and A. Oyama, “Evaluation of aerodynamic performance of Mars airplane in scientific balloon experiment,” Fluid Mech. Res. Int. J., Vol.1, No.3, Article No.00012, 2017. https://doi.org/10.15406/fmrij.2017.01.00012
- [11] D. Banfield, D. W. Schindel, S. Tarr, and R. W. Dissly, “A Martian acoustic anemometer,” J. Acoust. Soc. Am., Vol.140, No.2, pp. 1420-1428, 2016. https://doi.org/10.1121/1.4960737
- [12] J. W. Dankanich, T. Kremic, K. Hibbitts, E. F. Young, and R. Landis, “Planetary balloon-based science platform evaluation and program implementation: Final report,” NASA Technical Memorandum, No.NASA/TM-2016-218870, 2016.
- [13] A. Sóbester, H. Czerski, N. Zapponi, and I. Castro, “High-altitude gas balloon trajectory prediction: A Monte Carlo model,” AIAA J., Vol.52, No.4, pp. 832-842, 2014. https://doi.org/10.2514/1.J052900
- [14] R. Palumbo, G. Morani, and F. Corraro, “Effective approach to characterization of prediction errors for balloon ascent trajectories,” J. Aircr., Vol.47, No.4, pp. 1331-1337, 2010. https://doi.org/10.2514/1.47005
- [15] A. Smith, T. Fields, J. LaCombe, and E. Wang, “Development of a coupled dropsonde-autonomous descent vehicle system,” AIAA Aerodyn. Decelerator Syst. Conf., 2013. https://doi.org/10.2514/6.2013-1267
- [16] T. Fields, M. Heninger, J. LaCombe, and E. Wang, “In-flight landing location predictions using ascent wind data for high altitude balloons,” AIAA Balloon Syst. Conf., 2013. https://doi.org/10.2514/6.2013-1294
- [17] R. Farley, “BalloonAscent: 3-D simulation tool for the ascent and float of high-altitude balloons,” AIAA 5th ATIO and 16th Lighter-Than-Air Sys. Tech. and Balloon Syst. Conf., 2005. https://doi.org/10.2514/6.2005-7412
- [18] E. E. Riddle et al., “Trajectory model validation using newly developed altitude-controlled balloons during the International Consortium for Atmospheric Research on Transport and Transformations 2004 campaign,” J. Geophys. Res.: Atmos., Vol.111, No.D23, Article No.D23S57, 2006. https://doi.org/10.1029/2006JD007456
- [19] A. Gallice, F. G. Wienhold, C. R. Hoyle, F. Immler, and T. Peter, “Modeling the ascent of sounding balloons: Derivation of the vertical air motion,” Atmos. Meas. Tech., Vol.4, No.10, pp. 2235-2253, 2011. https://doi.org/10.5194/amt-4-2235-2011
- [20] S. Higashino, M. Hayashi, S. Nagasaki, S. Umemoto, and M. Nishimura, “A balloon-assisted gliding UAV for aerosol observation in Antarctica,” Trans. Jpn. Soc. Aeronaut. Space Sci. Aerosp. Technol. Jpn., Vol.12, No.APISAT-2013, pp. a35-a41, 2014. https://doi.org/10.2322/tastj.12.a35
- [21] N. Yajima, N. Izutsu, T. Imamura, and T. Abe, “Scientific Ballooning: Technology and Applications of Exploration Balloons Floating in the Stratosphere and the Atmospheres of Other Planets,” Springer, 2009.
- [22] D. J. Seidel, B. Sun, M. Pettey, and A. Reale, “Global radiosonde balloon drift statistics,” J. Geophys. Res.: Atmos., Vol.116, No.D7, Article No.D07102, 2011. https://doi.org/10.1029/2010JD014891
This article is published under a Creative Commons Attribution-NoDerivatives 4.0 Internationa License.