Paper:
Hydraulic Robotic Leg for HYDROïD Robot: Modeling and Control
Ahmed Abdellatif Hamed Ibrahim*1, Anas Ammounah*2, Samer Alfayad*2, Sami Tliba*3, Fethi Ben Ouezdou*4, and Stéphane Delaplace*5
*1Mechanical Engineering Department, Arab Academy for Science, Technology and Maritime Transport
El Mosheer Ahmed Ismael Street, Sheraton Al Matar, AASTMT Campus, El Nozha, Cairo 11799, Egypt
*2The Informatique, Bio-Informatique Systémes Complexes Laboratory
UFR Sciences et Technologies, 36 Rue du Pelvoux CE1455 Courcouronnes, Evry Cedex F-91020, France
*3CentraleSupélec, Université Paris-Saclay
Plateau de Moulon, 3 Rue Joliot-Curie, Gif-sur-Yvette Cedex F-91192, France
*4Vedecom institute
23 bis All. des Marronniers, Versailles 78000, France
*5University Institute of Technology at Vélizy-Villacoublay
10-12 Avenue de l’Europe, Vélizy 78140, France
This paper presents a new hydraulic robotic leg for the humanoid hydraulic robot HYDROïD. The main parts of this leg are divided into two main parts; the knee subsystem and the ankle subsystem. The presented leg mechanism consists of 4 DOFs, all of which are operated using highly dynamic servo valves. The design of the leg is thoroughly presented and all of its parts are demonstrated. Moreover, the inverse kinematics for both sub-mechanisms are presented to be able to control the angular position of the leg joints. Also, a virtual dynamic model is introduced in which a position controller is applied to the model to regulate the actuating pistons, check the new structure, and analyze the position of the joints and their torques. To test the new leg experimentally, the new leg is assembled with the rest of the robot, and rapid prototyping software is used with a position controller. Experimental position tracking responses are introduced showing the validity of the new design and the implemented controller.
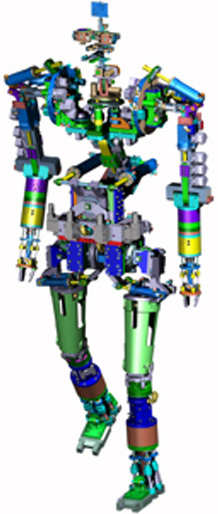
The new hydraulic leg for HYDROïD robot
- [1] S. Alfayad et al., “HYDROïD Humanoid Robot Head with Perception and Emotion Capabilities: Modeling, Design, and Experimental Results,” Frontiers in Robotics and AI, Vol.3, doi: 10.3389/frobt.2016.00015, 2016.
- [2] H. Kawasaki and T. Mouri, “Humanoid Robot Hand and its Applied Research,” J. Robot. Mechatron., Vol.31, No.1, pp. 16-26, 2019.
- [3] W.-F. Hsieh, E. Sato-Shimokawara, and T. Yamaguchi, “Investigation of Robot Expression Style in Human-Robot Interaction,” J. Robot. Mechatron., Vol.32, No.1, pp. 224-235, 2020.
- [4] Y. Hanazawa et al., “Analysis of Fast Bipedal Walking Using Mechanism of Actively Controlled Wobbling Mass,” J. Robot. Mechatron., Vol.31, No.6, pp. 871-881, 2019.
- [5] S. Tarao et al., “Prototyping Using a Mobile Robot Platform Equipped with Low-End In-Wheel Motors,” J. Robot. Mechatron., Vol.32, No.6, pp. 1154-1163, 2020.
- [6] K. H. Low et al., “Special Issue on Focused Areas and Future Trends of Bio-Inspired Robots “Analysis, Control, and Design for Bio-Inspired Robotics,” J. Robot. Mechatron., Vol.24, No.4, pp. 559-560, 2012.
- [7] JRM Staff Writer, “Autonomous Walking Humanoid that Astonished the World – Honda’s ASIMO,” J. Robot. Mechatron., Vol.26, No.1, pp. 15-16, 2014.
- [8] S. Ohta et al., “Improvement of Performance for Musculoskeletal Robots by Mountable Actuator Units,” J. Robot. Mechatron., Vol.22, No.3, pp. 391-401, 2010.
- [9] O. Stasse et al., “TALOS: A new humanoid research platform targeted for industrial applications,” IEEE-RAS 17th Int. Conf. on Humanoid Robotics (Humanoids), pp. 689-695, 2017.
- [10] Y. Nakanishi et al., “Design approach of biologically-inspired musculoskeletal humanoids,” Int. J. of Advanced Robotic Systems, Vol.10, Issue 4, 2013.
- [11] K. Kaneko et al., “Humanoid Robot HRP-5P: An Electrically Actuated Humanoid Robot with High-Power and Wide-Range Joints,” IEEE Robotics and Automation Letters, Vol.4, No.2, pp. 1431-1438, doi: 10.1109/LRA.2019.2896465, 2019.
- [12] H. Zhong et al., “Toward Safe Human-Robot Interaction: A Fast-Response Admittance Control Method for Series Elastic Actuator,” IEEE Trans. on Automation Science and Engineering, Vol.19, No.2, pp. 919-932, doi: 10.1109/TASE.2021.3057883, 2022.
- [13] K. Suzumori, “New Robotics Pioneered by Fluid Power,” J. Robot. Mechatron., Vol.32, No.5, pp. 854-862, 2020.
- [14] N. Rotella et al., “Inertial sensor-based humanoid joint state estimation,” IEEE Int. Conf. on Robotics and Automation (ICRA), pp. 1825-1831, doi: 10.1109/ICRA.2016.7487328, 2016.
- [15] T. Ko et al., “Compliant Biped Locomotion of Hydra, an Electro-Hydrostatically Driven Humanoid,” IEEE-RAS 18th Int. Conf. on Humanoid Robots (Humanoids), pp. 280-283, doi: 10.1109/HUMANOIDS.2018.8624973, 2018.
- [16] K. Hirayama, N. Hirosawa, and S. Hyon, “Passivity-Based Compliant Walking on Torque-Controlled Hydraulic Biped Robot,” IEEE-RAS 18th Int. Conf. on Humanoid Robots (Humanoids), doi: 10.1109/HUMANOIDS.2018.8624964, 2018.
- [17] F. Gao et al., “A quadruped robot with parallel mechanism legs,” IEEE Int. Conf. on Robotics and Automation (ICRA), p. 2566, 2014.
- [18] S. Bertrand et al., “Detecting Usable Planar Regions for Legged Robot Locomotion,” IEEE/RSJ Int. Conf. on Intelligent Robots and Systems (IROS), pp. 4736-4742, doi: 10.1109/IROS45743.2020.9341000, 2020.
- [19] A. Abdellatif, S. Hallak, and S. Alfayad, “Development of an On-Board Power Pack for the Hydraulic Humanoid Robot HYDROïD,” Int. Review of Mechanical Engineering (IREME), Vol.12, No.8, pp. 726-733, 2018.
- [20] B. Cho et al., “Design of a Compact Embedded Hydraulic Power Unit for Bipedal Robots,” IEEE Robotics and Automation Letters, Vol.6, Issue 2, pp. 3631-3638, doi: 10.1109/LRA.2021.3061390, 2021.
- [21] Z. Hua et al., “Analysis and verification on energy consumption of the quadruped robot with passive compliant hydraulic servo actuator,” Applied Science, Vol.10, No.1, Article No.340, 2020.
- [22] M. Dong et al., “State of the art in parallel ankle rehabilitation robot: a systematic review,” J. NeuroEngineering Rehabil., Vol.18, Article No.52, doi: 10.1186/s12984-021-00845-z, 2021.
- [23] G. Ficht and S. Behnke, “Bipedal Humanoid Hardware Design: A Technology Review,” Curr. Robot. Rep., Vol.2, pp. 201-210, doi: 10.1007/s43154-021-00050-9, 2021.
- [24] S. Hyon et al., “Development of a fast torque-controlled hydraulic humanoid robot that can balance compliantly,” IEEE-RAS 15th Int. Conf. on Humanoid Robots (Humanoids), pp. 576-581, doi: 10.1109/HUMANOIDS.2015.7363420, 2015.
- [25] G. Cheng et al., “CB: A Humanoid Research Platform for Exploring NeuroScience,” 6th IEEE-RAS Int. Conf. on Humanoid Robots, pp. 182-187, doi: 10.1109/ICHR.2006.321382, 2006.
- [26] Y. Lei et al., “Mechanical design and gait plan of a hydraulic-actuated biped robot,” IEEE Int. Conf. on Mechatronics and Automation (ICMA), pp. 1132-1137, doi: 10.1109/ICMA.2015.7237645, 2015.
- [27] M. Elasswad et al., “Development of lightweight hydraulic cylinder for humanoid robots applications” Proc. of the Institution of Mechanical Engineers, Part C: J. of Mechanical Engineering Science, Vol.232, No.18, pp. 3351-3364, doi: 10.1177/0954406217731794, 2017.
- [28] S. Alfayad, F. B. Ouezdou, and F. Namoun, “New 3-DOFs Hybrid Mechanism for Ankle and Wrist of Humanoid Robot: Modeling, Simulation, and Experiments,” J. Mech. Des., Vol.133, Issue 2, Article No.021005, 2011.
- [29] A. Abdellatif et al., “Development of a new hydraulic ankle for HYDROïD humanoid robot,” J. of Intelligent & Robotic Systems, Vol.92, pp. 293-308, 2018.
- [30] K. Narioka, T. Homma, and K. Hosoda, “Humanlike ankle-foot complex for a biped robot,” IEEE-RAS Int. Conf. on Humanoid Robots (Humanoids), pp. 15-20, 2012.
- [31] J. Kim et al., “Development of the lower limbs for a humanoid robot,” IEEE/RSJ Int. Conf. Intell. Robot. Syst., pp. 4000-4005, 2012.
- [32] J. Luo, Y. Fu, and S. Wang, “Design and Walking Control Implementation of a Hydraulic Humanoid Biped Robot,” Int. J. Mech. Eng. Autom., Vol.4, No.2, pp. 15-25, 2017.
- [33] E. M. Ficanha et al., “Ankle Angles During Step Turn and Straight Walk: Implications for the Design of a Steerable Ankle-Foot Prosthetic Robot,” Proc. ASME 2013 Dyn. Syst. Control Conf., Vol.1, doi: 10.1115/DSCC2013-3782, 2013.
- [34] K. Hashimoto, “Mechanics of Humanoid Robot, Advanced Robotics,” Advanced Robotics, Vol.34, No.21-22, pp. 1390-1397, doi: 10.1080/01691864.2020.1813624, 2020.
- [35] P. Long, W. Khalil, and P. Martinet, “Dynamic modeling of parallel robots with flexible platforms,” Mech. Mach. Theory, Vol.81, pp. 21-35, 2014.
- [36] J. Chen, X. Mu, and F. Du, “Biomechanics analysis of human lower limb during walking for exoskeleton design,” J. Vibroengineering, Vol.19, Issue 7, pp. 5527-5539, 2017.
- [37] C. Vasileiou et al., “Development of a passive biped robot digital twin using analysis, experiments, and a multibody simulation environment,” Mech. Mach. Theory, Vol.163, Article No.104346, doi: 0.1016/j.mechmachtheory.2021.104346, 2021.
This article is published under a Creative Commons Attribution-NoDerivatives 4.0 Internationa License.