Paper:
Supporting Effects on Muscles of a Motion Assistive Wear Depending on the Fixture Position
Kazunori Ogawa*1,*2, Akito Kadowaki*1, Koji Shimatani*3, Masaki Hasegawa*3, Keita Takahashi*1, Toshio Tsuji*1, and Yuichi Kurita*1,*4
*1Graduate School of Engineering, Hiroshima University
1-4-1 Kagamiyama, Higashihiroshima, Hiroshima 739-8527, Japan
*2Daiya Industries Co., Ltd.
1125 Koshinden, Minami-ku, Okayama-shi, Okayama 701-0203, Japan
*3Prefectural University of Hiroshima
1-1 Gakuen-machi, Mihara City, Hiroshima 723-0053, Japan
*4PRESTO (Sakigake), Japan Society and Technology Agency (JST)
7 Gobancho, Chiyoda-ku, Tokyo 102-0076, Japan
As muscle weakness due to aging or fatigue potentially increases the risk of injuries or accidents, support wears may be needed that can play a role in assisting various motions. Recently, research and development of such support wears have gained momentum in order to measure a variety of their supporting effects. While in the research stage, they are designed and evaluated on the premise that they are carefully adjusted to fit the specific wearers’ physiques. However, in actually producing and using such support wears on sites, they pose a problem in that their supporting effects cannot sufficiently be felt because they are difficult to fix, or because they do not fit all body sizes. Therefore, in this study, we have quantitatively evaluated their assisting effects using simulations with a musculoskeletal model with a built-in functional underwear with support parts. In particular, we evaluated whether any differences in arrangement of support parts on the human body, due to their different fixture methods, will have an effect on their assisting effects. We have also developed a support wear, with easy to adjust support parts and fixture positions, and have verified the abovementioned simulation accuracies by myoelectric measurements. This verification found that the proposed simulation method can predict, to some extent, the impacts of any deviations in the support parts’ positions and their assisting effects.
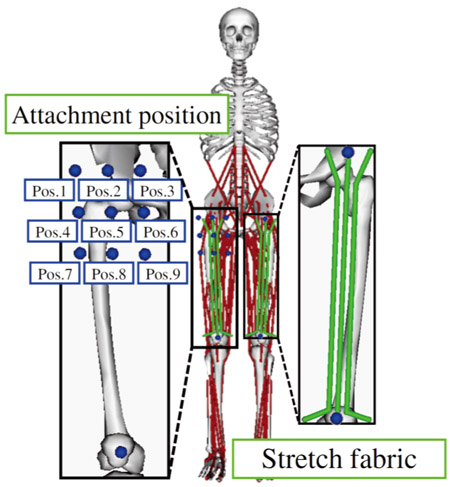
Supporting effects on muscles of a motion assistive wear
- [1] H. Kawamoto and Y. Sankai, “Power assist system HAL-3 for gait disorder person,” Int. Conf. on Computers for Handicapped Persons, pp. 196-203, 2002.
- [2] K. Yamamoto, K. Hyodo, M. Ishii, and T. Matsuo, “Development of power assisting suit for assisting nurse labor,” JSME Int. J. Series C Mechanical Systems, Machine Elements and Manufacturing, Vol.45, No.3, pp. 703-711, 2002.
- [3] H. Wu, A. Kitagawa, H. Tsukagoshi, and C. Liu, “Development of a novel pneumatic power assisted lower limb for outdoor walking by the use of a portable pneumatic power source,” 2007 IEEE Int. Conf. on Control Applications, pp. 1291-1296, 2007.
- [4] S. Toyama and J. Yonetake, “Development of the ultrasonic motor-powered assisted suit system,” IEEE/ICME Int. Conf. on Complex Medical Engineering, pp. 1361-1366, 2007.
- [5] Y. Ming, Z. Meiling, R. C. Richardson, M. C. Levesley, P. G. Walker, and K. Watterson, “Design and evaluation of linear ultrasonic motors for a cardiac com-pression assist device,” Sensors and Actuators A: Physical, Vol.119, No.1, pp. 214-220, 2005.
- [6] T. Noritsugu, D. Sasaki, M. Kameda, A. Fukunaga, and M. Takaiwa, “Wearable power assist device for standing up motion using pneumatic rubber artificial muscles,” J. Robot. Mechatron., Vol.19, No.6, pp. 619-628, 2007.
- [7] M. Aragane, T. Noritsugu, M. Takaiwa, D. Sasaki, and S. Naomoto, “Development of sheet-like curved type pneumatic rubber muscle and application to elbow power assist wear,” J. of the Robotics Society of Japan, Vol.26, No.6, pp. 674-682, 2008.
- [8] N. Yamazaki and N. Takahashi, “Suit-type Back Muscle Aupporter Utilizing Body Surface Deformation During Care Motions,” Society of Biomechanisms Japan, Vol.17, pp. 235-244, 2004.
- [9] T. Okazaki and H. Yokoi, “The Approach of Arrangement Decision Problem of Highly-Elastic Fabric for Motion Support,” Proc. of the 27th Annual Conf. of the Robotics Society of Japan, 1C3-05, 2009.
- [10] Y. Imamura, T. Tanaka, Y. Suzuki, K. Takizawa, and M. Yamanaka, “Motion-Based-Design of Elastic Material for Passive Assistive Device Using Musculoskeletal Model,” J. Robot. Mechatron., Vol.23, No.6, pp. 978-990, 2011.
- [11] H. Inoue and T. Noritsugu, “Development of Upper-Limb power assist machine using linkage mechanism – Drive mechanism and its applications –,” J. Robot. Mechatron., Vol.30, No.2, pp. 214-222, 2018.
- [12] Y. Hasegawa, M. Tayama, T. Saito, and Y. Sankai, “Active air mat for comfortable and easy to wear a forearm support system,” 2011 IEEE/RSJ Int. Conf. on Intelligent Robots and Systems, pp. 4899-4904, 2011.
- [13] M. Higuchi and T. Ogasawara, “Development of a Human Symbiotic Assist Arm “PAS-Arm”,” J. of the Robotics Society of Japan, Vol.25, No.2, pp. 285-293, 2013.
- [14] Y. Endo, S. Kanai, N. Miyata, M. Kouchi, M. Mochimaru, J. Konno, and M. Shimokawa, “Optimization-based grasp posture generation method of digital hand for virtual ergonomics assessment,” SAE Int. J. of Passenger Cars-Electronic and Electrical Systems, Vol.1, No.2008-01-1902, pp. 590-598, 2008.
- [15] Y. Muramatsu, H. Umehara, and H. Kobayashi, “Improvement and quantitative performance estimation of the back support muscle suit,” 2013 35th Annual Int. Conf. of the IEEE Engineering in Medicine and Biology Society (EMBC), pp. 2844-2849, 2013.
- [16] S. L. Delp, J. P. Loan, M. G. Hoy, F. E. Zajac, E. L. Topp, and J. M. Rosen, “An interactive graphics-based model of the lower extremity to study orthopaedic surgical procedures,” IEEE Trans. on Biomedical Engineering, Vol.37, pp. 757-767, 1990.
- [17] M. Christophy, N. A. F. Senan, J. C. Lotz, and O. M. O’Reilly, “Musculoskeletal model for the lumbar spine,” Biomech Model Mechanobiol, Vol.11, Nos.1-2, pp. 19-34, 2012.
- [18] S. L. Delp, F. C. Anderson, A. S. Arnold, P. Loan, A. Habib, C. T. John, E. Guendelman, and D. G. Thelen, “OpenSim: Open-source Software to Create and Analyze Dynamic Simulations of Movement,” IEEE Trans. on Biomedical Engineering, Vol.54, No.11, pp. 1940-1950, 2007.
- [19] D. G. Thelen, “Adjustment of muscle mechanics model parameters to simulate dynamic contractions in older adults,” J. of Biomechanical Engineering, Vol.125, No.1, pp. 70-77, 2003.
- [20] R. D. Crowninshield and R. A. Brand, “A physiologically based criterion of muscle force prediction in locomotion,” J. of Biomechanics, Vol.14, No.11, pp. 793-801, 1981.
- [21] W. Maurel and D. Thalmann, “A Case Study Analysis on Human Upper Limb Modeling for Dynamic Simulation,” J. of Computer Methods in Biomechanics and Biomedical Engineering, Vol.2, No.1, pp. 65-82, 1999.
This article is published under a Creative Commons Attribution-NoDerivatives 4.0 Internationa License.