Paper:
Fluid-Structure Interaction Analysis of a Soft Robotic Fish Using Piezoelectric Fiber Composite
Wenjing Zhao, Aiguo Ming, Makoto Shimojo,
Yohei Inoue, and Hiroshi Maekawa
Department of Mechanical Engineering and Intelligent Systems, The University of Electro-Communications, 1-5-1 Chofugaoka, Chofu-shi, Tokyo 182-8585, Japan
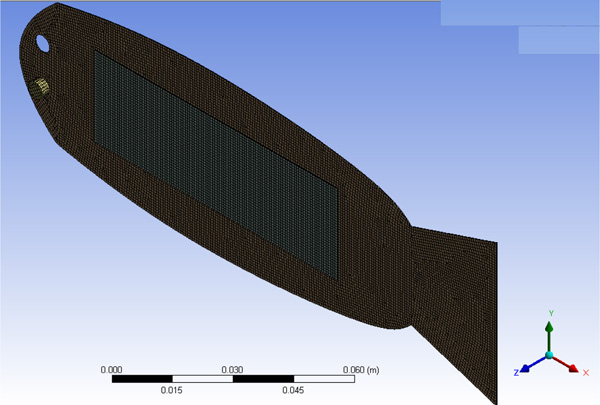
- [1] E. Kim and Y. Youm, “Design and dynamic analysis of fish robot: PoTuna,” Proc. of IEEE Int. Conf. on Robotics and Automation, pp. 4887-4892, 2004.
- [2] J.M. Anderson, “The vorticity control unmanned undersea vehicle,” Proc. of Int. Symposium on Seawater Drag Reduction, pp. 479-483, 1998.
- [3] X. Deng and S. Avadhanula, “Biomimetic micro underwater vehicle with oscillating fin propulsion: system design and force measurement,” Proc. of IEEE Int. Conf. on Robotics and Automation, pp. 3312-3317, 2005.
- [4] J. M. Kumph, “Maneuvering of a robotic pike,” M. S. Thesis, Massachusetts Institute of Technology, USA, 2000.
- [5] M. S. Triantafyllou, A. H. Techet, and F. S. Hover, “Review of experiment work in biomimetic foils,” IEEE J. of Oceanic Engineering, Vol.29, No.3, pp. 585-593, 2004.
- [6] K. H. Low, C.W. Chong, and C. Zhou, “Performance study of a fish robot propelled by a flexible caudal fin,” Proc. of IEEE Int. Conf. on Robotics and Automation, pp. 90-95, 2010.
- [7] X. Tan, M. Carpenter, J. Thon, and F. Alequin-Ramos, “Analytical modeling and experimental studies of robotic fish turning,” Proc. of IEEE Int. Conf. on Robotics and Automation, pp. 102-108, 2010.
- [8] A. Crespi, A. Badertscher, A. Guignard, and A. J. Iispeert, “Amphi-Bot I: an amphibious snake-like robot,” Robotics and Autonomous Systems, Vol.50, pp. 163-175, 2005.
- [9] H. Hu, “Biologically inspired design of autonomous robotic fish at Essex,” Proc. of IEEE SMC UK-RI Chapter Conf. on Advances in Cybernetic Systems, pp. 1-8, 2006.
- [10] S. Hirose and E. F. Fukushima, “Snakes and strings: new robotic components for rescue operations,” Int. J. of Robotics Research, Vol.23, pp. 341-349, 2004.
- [11] M. Mojarrad and M. Shahinpoor, “Biomimetic robotic propulsion using polymeric artificial muscles,” Proc. of IEEE Int. Conf. on Robotics and Automation, pp. 2152-2157, 1997.
- [12] Z. Zhang, N. Yamashita, and M. Gondo, “Electrostatically actuated robotic fish: design and control for high-mobility open-loop swimming,” IEEE Trans. on Robotics, Vol.24, No.1, pp. 118-129, 2008.
- [13] S. Guo, Y. Ge, L. Li, and S. Liu, “Underwater swimming micro robot using IPMC actuator,” Proc. of IEEE Int. Conf. on Mechatronics and Automation (ICMA), pp. 249-254, 2006.
- [14] O. K. Rediniotis, L. N. Wilson, D. C. Lagoudas, and M. M. Khan, “Development of a Shape-Memory-Alloy actuated biomimetic hydrofoil,” J. of Intelligent Material Systems and Structures, Vol.13, No.1, pp. 35-49, 2002.
- [15] T. Fukuda and H. Hosokai, “Giant magnetostrictive alloy (GMA) application to micro mobile robot as a micro actuator without power supply cable,” Proc. of IEEE Int. Conf. on Micro Electro Mechanical System (MEMS), pp. 210-215, 1991.
- [16] R. B. Williams and D. J. Inman, “An overview of composite actuators with piezoceramic fibers,” Proc. of 20th Int. Modal Analysis Conf., pp. 421-427, 2002.
- [17] K. H. Low and A. Willy, “Biomimetic motion planning of an undulating robotic fish fin,” J. of Vibration and Control, Vol.12, No.12, pp. 1337-1359, 2006.
- [18] L. Jia and X. Yin, “Passive oscillations of two tandem flexible filaments in a flowing soap film,” Physical review letters, Vol.100, No.22, pp. 1-4, 2008.
- [19] D. Xia, J. Liu, W. Cheng, and L. Han, “Numerical simulation of the hydrodynamics of fishlike robot swimming based on converged speed,” J. of Mechanical Engineering, Vol.46, No.1, pp. 48-54, 2010.
- [20] T. Y. Wu, “Hydrodynamics of swimming propulsion. Part 1. Swimming of a two-dimensional flexible plate at variable forward speeds in an inviscid fluid,” J. of Fluid Mechanics, Vol.46, No.2, pp. 337-355, 1971.
- [21] M. Lighthill, “Large amplitude elongated-body theory of fish locomotion,” Pro. R. Soc. London, Ser. B, Vol.179, pp. 125-138, 1971.
- [22] J. N. Newman, “The force on a slender fish-like body,” J. of Fluid Mechanics, Vol.58, No.4, pp. 689-702, 1973.
- [23] J. Cheng, L. Zhuang, and B. Tong, “Analysis of swimming three-dimensional waving plates,” J. of Fluid Mechanics, Vol.232, pp. 341-355, 1991.
- [24] H. Kagemoto, M. J. Wolfgang, D. K. P. Yue, and M. S. Triantafyllou, “Force and power estimation in fish-like locomotion using a vortex-lattice method,” J. of Fluids Engineering, Vol.122, No.2, pp. 239-253, 2000.
- [25] H. Liu and K. Kawachi, “A numerical study of undulatory swimming,” J. Comput. Phys., Vol.155, No.2, pp. 223-247, 1999.
- [26] H.-J. Bungartz and M. Schäfer, “Fluid-structure interaction: modelling, simulation, optimization,” Springer-Verlag, 2006.
- [27] W. Dettmer and D. Peric, “A computational framework for fluidstructure interaction: finite element formulation and applications,” Computer Methods in Applied Mechanics and Engineering, Vol.195, pp. 5754-5779, 2006.
- [28] S. Gong, L. Ren, H. Liu, L. Li, and Z. Zou, “Fluid-solid interaction of rotor blade in last stage of steam turbine,” Thermal Turbine, Vol.36, No.3, pp. 153-157, 2007.
- [29] D. Tang, C. Yang, S. Kobayashi, and D. N. Ku, “Effect of a lipid pool on stress/strain distributions in stenotic arteries: 3-D fluid–structure interactions (FSI) models,” J. Biomech. Eng., Vol.126, No.3, pp. 363-370, 2004.
- [30] D. Tang, C. Yang, S. Mondal, F. Liu, G. Canton, T. Hatsukami, and C. Yuan, “A negative correlation between human carotid atherosclerotic plaque progression and plaque wall stress: in vivo MRI-based 2D/3D FSI models,” J. Biomech., Vol.41, No.4, pp. 727-736, 2008.
- [31] J. Leach, V. Rayz, M. Mofrad, and D. Saloner, “An efficient twostage approach for image-based FSI analysis of atherosclerotic arteries,” Biomech. Model Mechanobiol, Vol.9, No.2, pp. 213-223, 2010.
- [32] H.-J. Bungartz, M. Mehl, and M. Schäfer, “Fluid-structure interaction II: modelling, simulation, optimization,” Springer-Verlag, 2010.
- [33] H. Schmucker, F. Flemming, and S. Coulson, “Two-way coupled fluid structure interaction simulation of a propeller turbine,” The 25th IAHR Symposium on Hydraulic Machinery and Systems/ IOP Conf. Series: Earth and Environmental Science, Vol.12, No.1, pp. 1-10, 2010.
- [34] R. L. Campbell, “Fluid-structure interaction and inverse design simulations for flexible turbomachinery,” Doctoral Dissertation of the Pennsylvania State University, 2010.
- [35] M. Hsu and Y. Bazilevs, “Fluid-structure interaction modeling of wind turbines: simulating the full machine,” Computational Mechanics, Vol.50, No.6, pp. 821-833, 2012.
- [36] R. B. Williams, “Nonlinear mechanical and actuator characterization of piezoelectric fiber composites,” Doctoral Dissertation of Virginia Polytechnic Institute and State University, 2004.
- [37] J. W. High and W. K. Wilkie, “Method of fabricating NASA-standard macro-fiber composite piezoelectric actuators,” NASA/TM-2003-212427, Langley Research Center, Hampton, Virginia, 2003.
- [38] ANSYS Inc., “ANSYS theory reference 12.1,” ANSYS Inc., 2009.
- [39] I. Borazjani and F. Sotiropoulos, “Numerical investigation of the hydrodynamics of carangiform swimming in the transitional and inertial flow regimes,” J. of Experimental Biology, Vol.211, pp. 1541-1558, 2008.
- [40] I. Tanaka and M. Nagai, “Hydrodynamic of resistance and propulsion-learn from the fast swimming ability of aquatic animals,” Ship & Ocean Foundation, pp. 14-19, 1996.
- [41]
Supporting Online Materials:[a] Smart Material, 2012.
http://www.smart-material.com [Accessed February 2012]
This article is published under a Creative Commons Attribution-NoDerivatives 4.0 Internationa License.