Paper:
Motion Evaluation of Variable-Stiffness Link Based on Shape-Memory Alloy and Jamming Transition Phenomenon
Kazuto Takashima*
, Hidetaka Suzuki*, Toshiki Imazawa*, and Hiroki Cho**
*Graduate School of Life Science and Systems Engineering, Kyushu Institute of Technology
2-4 Hibikino, Wakamatsu-ku, Kitakyushu, Fukuoka 808-0196, Japan
**Faculty of Environmental Engineering, The University of Kitakyushu
1-1 Hibikino, Wakamatsu-ku, Kitakyushu, Fukuoka 808-0135, Japan
In rapidly aging societies, the application of robots has spread from industry to nursing and social welfare. As the designs of industrial and non-industrial robots are different, numerous robot components with various shapes and stiffness are required for different tasks. In this study, we attached a variable-stiffness link based on a shape-memory alloy (SMA) and the jamming transition phenomenon to a robot arm and evaluated its pick-and-place motion for various objects with different shapes and weights. The link can be fixed in an arbitrary shape and then restored to its initial shape via the shape memory effect. The objects were picked up and moved by a prototype link, which consisted of four SMA wires inserted in the jamming mechanism. We compared two states of the link, namely with and without deformation of the link into a shape (the centerline and the cross section) to suit the target object using a mold. Experiments confirmed that changing and fixing the link shape to suit the target object increased both positioning accuracy and weight capacity.
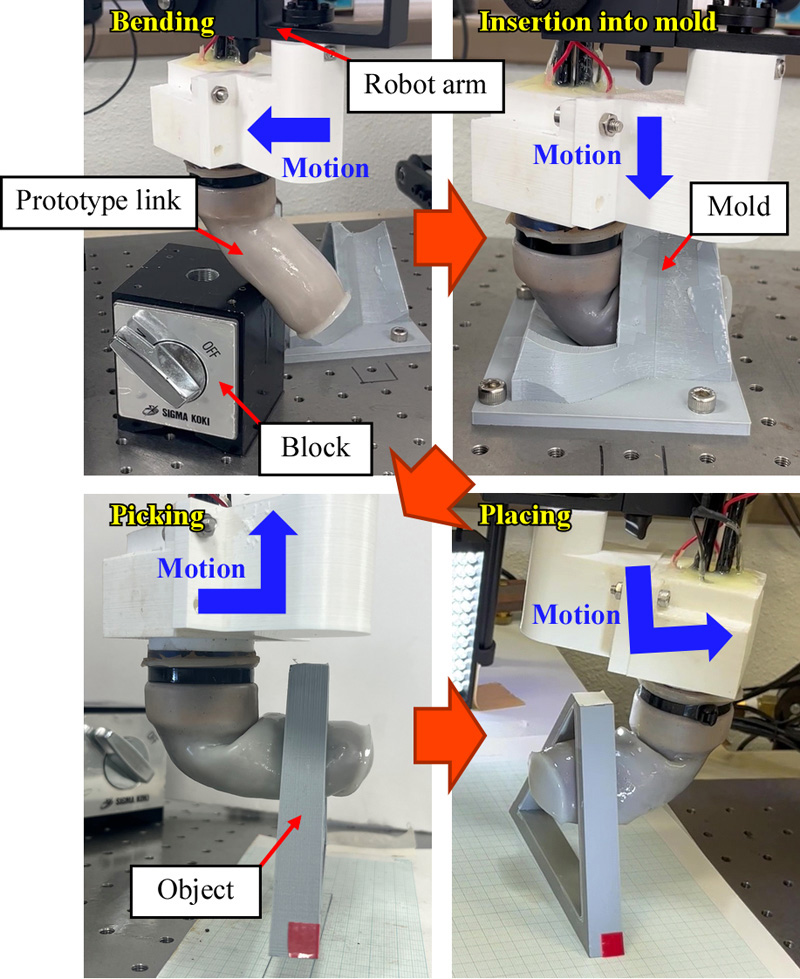
Pick-and-place motion of link
- [1] T. Liu, H. Xia, D.-Y. Lee, A. Firouzeh, Y. -L. Park, and K.-J. Cho, “A positive pressure jamming based variable stiffness structure and its application on wearable robots,” IEEE Robotics and Automation Letters, Vol.6, No.4, pp. 8078-8085, 2021. https://doi.org/10.1109/LRA.2021.3097255
- [2] S. Wolf, G. Grioli, O. Eiberger, W. Friedl, M. Grebenstein, H. Höppner, E. Burdet, D. Caldwell, R. Carloni, M. Catalano, D. Lefeberk, S. Stramigioli, N. Tsagarakis, M. van Dammek, R. van Hamk, B. Vanderborghtk, L. C. Visser, A. Bicchi, and A. Albu-Schäffer, “Variable stiffness actuators: Review on design and components,” IEEE/ASME Trans. on Mechatronics, Vol.21, No.5, pp. 2418-2430, 2016. https://doi.org/10.1109/TMECH.2015.2501019
- [3] J. Shintake, V. Cacucciolo, D. Floreano, and H. Shea, “Soft robotic grippers,” Adv. Mater., Vol.30, Article No.1707035, 2018. https://doi.org/10.1002/adma.201707035
- [4] D. Rus and M. T. Tolley, “Design, fabrication and control of soft robots,” Nature, Vol.521, No.7553, pp. 467-475, 2015. https://doi.org/10.1038/nature14543
- [5] J. Hughes, U. Culha, F. Giardina, F. Guenther, A. Rosendo, and F. Iida, “Soft manipulators and grippers: A review,” Front. Robot. AI, Vol.3, Article No.69, 2016. https://doi.org/10.3389/frobt.2016.00069
- [6] N. G. Cheng, M. B. Lobovsky, S. J. Keating, A. M. Setapen, K. I. Gero, A. E. Hosoi, and K. D. Iagnemma, “Design and analysis of a robust, low-cost, highly articulated manipulator enabled by jamming of granular media,” Proc. of IEEE Int. Conf. on Robotics and Automation (ICRA), pp. 4328-4333, 2012. https://doi.org/10.1109/ICRA.2012.6225373
- [7] E. Steltz, A. Mozeika, N. Rodenberg, E. Brown, and H. M. Jaeger, “JSEL: Jamming skin enabled locomotion,” Proc. of the 2009 IEEE/RSJ Int. Conf. on Intelligent Robots and Systems, pp. 5672-5677, 2009. https://doi.org/10.1109/IROS.2009.5354790
- [8] E. Brown, N. Rodenberg, J. Amend, A. Mozeika, E. Steltz, M. R. Zakin, H. Lipson, and H. M. Jaeger, “Universal robotic gripper based on the jamming of granular material,” Proc. Natl. Acad. Sci. U.S.A., 2010. https://doi.org/10.1073/pnas.1003250107
- [9] A. Jiang, P. Dasgupta, K. Althoefer, and T. Nanayakkara, “Robotic granular jamming: A new variable stiffness mechanism,” J. of the Robotics Society of Japan, Vol.32, pp. 333-338, 2014. https://doi.org/10.7210/jrsj.32.333
- [10] T. Nishida, D. Shigehisa, N. Kawashima, and K. Tadakuma, “Development of universal jamming gripper with a force feedback mechanism,” Proc. of 7th Int. Conf. on Soft Computing and Intelligent Systems (SCIS) and 15th Int. Symp. on Advanced Intelligent Systems (ISIS), pp. 242-246, 2014. https://doi.org/10.1109/SCIS-ISIS.2014.7044693
- [11] S. Yamane and S. Wakimoto, “Development of a flexible manipulator with changing stiffness by granular jamming,” Proc. of 24th Int. Conf. on Mechatronics and Machine Vision in Practice (M2VIP), pp. 1-5, 2017. https://doi.org/10.1109/M2VIP.2017.8211491
- [12] Z. Hu, A. Ahmed, W. Wan, T. Watanabe, and K. Harada, “A stiffness-changeable soft finger based on chain mail jamming,” Proc. of 2023 IEEE Int. Conf. on Robotics and Automation (ICRA), pp. 7405-7411, 2023. https://doi.org/10.1109/ICRA48891.2023.10161061
- [13] M. Yamano, N. Akiba, J. Gong, and H. Furukawa, “Experiments of a two-arm robot using shape memory gel,” Proc. of IEEE/SICE Int. Symposium on System Integration (SII), pp. 236-241, 2012. https://doi.org/10.1109/SII.2012.6426947
- [14] H. Nakai, Y. Hoshino, M. Inaba, and H. Inoue, “Softening deformable robot: Development of shape adaptive robot using phase change of low-melting-point alloy,” J. of the Robotics Society of Japan, Vol.20, pp. 625-630, 2002 (in Japanese). https://doi.org/10.7210/jrsj.20.625
- [15] A. Miriyev, K. Stack, and H. Lipson, “Soft material for soft actuators,” Nat. Commun., Vol.8, Article No.596, 2017. https://doi.org/10.1038/s41467-017-00685-3
- [16] K. Suzumori, “Overview of the Kakenhi Grant-in-Aid for Scientific Research on Innovative Areas: Science of Soft Robots,” J. Robot. Mechatron., Vol.34, No.2, pp. 195-201, 2022. https://doi.org/10.20965/jrm.2022.p0195
- [17] J. Shintake, “Green robotics: Toward realization of environmentally friendly soft robots,” J. Robot. Mechatron., Vol.34, No.2, pp. 270-272, 2022. https://doi.org/10.20965/jrm.2022.p0270
- [18] J. Tanaka, “Vacuum end effector equipped with an expansion and contraction mechanism using a wound thin metal plate,” J. Robot. Mechatron., Vol.34, No.2, pp. 430-443, 2022. https://doi.org/10.20965/jrm.2022.p0430
- [19] K. Takashima, D. Iwamoto, S. Oshiro, T. Noritsugu, and T. Mukai, “Characteristics of pneumatic artificial rubber muscle using two shape-memory polymer sheets,” J. Robot. Mechatron., Vol.33, No.3, pp. 653-664, 2021. https://doi.org/10.20965/jrm.2021.p0653
- [20] K. Takashima, K. Sugitani, N. Morimoto, S. Sakaguchi, T. Noritsugu, and T. Mukai, “Pneumatic artificial rubber muscle using shape-memory polymer sheet with embedded electrical heating wire,” Smart Mater. Struct., Vol.23, No.12, Article No.125005, 2014. https://doi.org/10.1088/0964-1726/23/12/125005
- [21] K. Takashima, J. Rossiter, and T. Mukai, “McKibben artificial muscle using shape-memory polymer,” Sensors & Actuators: A Physical, Vol.164, pp. 116-124, 2010. https://doi.org/doi.org/10.1016/j.sna.2010.09.010
- [22] K. Takashima, T. Noritsugu, J. Rossiter, S. Guo, and T. Mukai, “Curved type pneumatic artificial rubber muscle using shape-memory polymer,” J. Robot. Mechatron., Vol.24, No.3, pp. 472-479, 2012. https://doi.org/10.20965/jrm.2012.p0472
- [23] K. Takashima, H. Kamizono, M. Takenaka, and T. Mukai, “Force sensor utilizing stiffness change of shape-memory polymer based on temperature,” ROBOMECH J., Vol.4, Article No.17, 2017. https://doi.org/10.1186/s40648-017-0086-2
- [24] K. Takashima, R. Miyazaki, and T. Mukai, “Surface shape changeable tactile sensor using shape-memory polymer,” Proc. of the SICE Annual Conf. 2021, pp. 1166-1171, 2021.
- [25] K. Takashima, T. Imazawa, and H. Cho, “Variable-stiffness and deformable link using shape-memory material and jamming transition phenomenon,” J. Robot. Mechatron., Vol.34, No.2, pp. 466-477, 2022. https://doi.org/10.20965/jrm.2022.p0466
- [26] J. M. Jani, M. Leary, A. Subic, and M. A. Gibson, “A review of shape memory alloy research, applications and opportunities,” Mater. Des., Vol.56, pp. 1078-1113, 2014. https://doi.org/10.1016/j.matdes.2013.11.084
- [27] Y. Haga, T. Mineta, T. Matsunaga, and N. Tsuruoka, “Micro-robotic medical tools employing SMA actuators for use in the human body,” J. Robot. Mechatron., Vol.34, No.6, pp. 1233-1244, 2022. https://doi.org/10.20965/jrm.2022.p1233
- [28] H. Cho, T. Yamamoto, Y. Takeda, A. Suzuki, and T. Sakuma, “Exploitation of shape memory alloy actuator using resistance feedback control and its development,” Prog. Nat. Sci., Vol.20, pp. 97-103, 2010. https://doi.org/10.1016/S1002-0071(12)60013-6
- [29] B. Calli, A. Singh, J. Bruce, A. Walsman, K. Konolige, S. Srinivasa, P. Abbeel, and A. M. Dollar, “Yale-CMU-Berkeley dataset for robotic manipulation research,” Int. J. Rob. Res., Vol.36, pp. 261-268, 2017. https://doi.org/doi.org/10.1177/0278364917700714
This article is published under a Creative Commons Attribution-NoDerivatives 4.0 Internationa License.