Paper:
Automatic Design of Serial Linkage Using Virtual Screw Joint
Atsushi Takata

Tokyo University of Agriculture and Technology
2-24-16 Naka-cho, Koganei-shi, Tokyo 184-8588, Japan
Here, an automatic design method for a serial link mechanism is proposed. This method outputs all kinematic parameters of joints, position, orientation, number, and type of joint (revolute or prismatic). Several studies have been conducted on optimizing only the positions and directions of joints for a desired path. However, automatically determining the numbers and types of joints requires an excessive calculation time owing to the complicity of kinematics. To handle heavy computation, a virtual screw joint (VSJ) is introduced based on a screw axis and the product of exponentials formula. Screw joints have the advantage of including both rotation and translation. First, an additional joint is optimized as a VSJ. Then, adopting its position and orientation and selecting a revolute or prismatic joint facilitate an efficient design process. To demonstrate the effectiveness of this study, two task motions addressed in a related work are adopted as target paths. Consequently, the proposed method automatically generates serial linkages that contain both revolute and prismatic joints and can follow along desired paths.
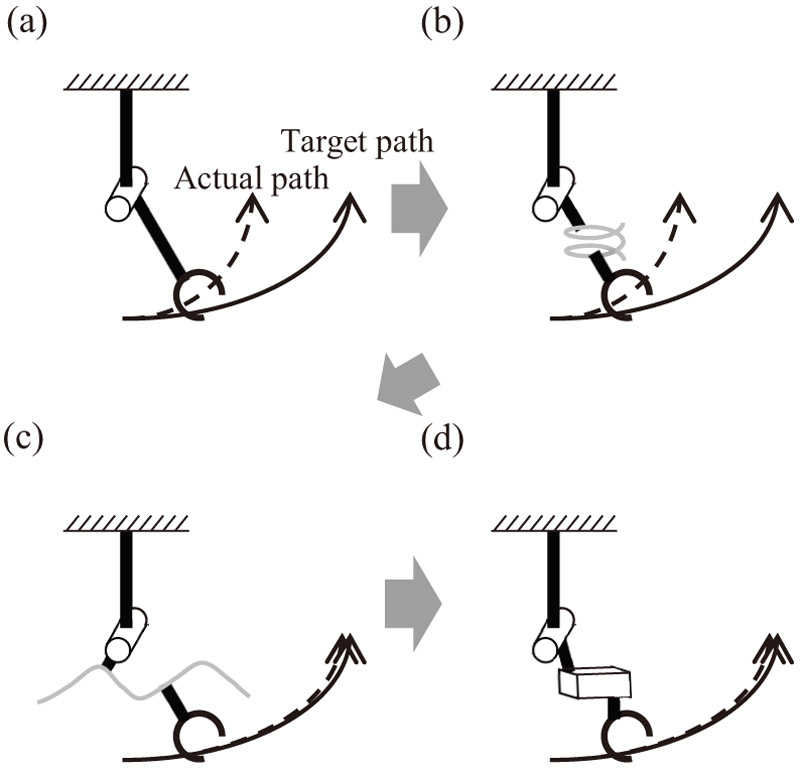
Automatic design method with virtual screw joint
- [1] K. Ueda, H. Yamada, H. Ishida, and S. Hirose, “Design of Large Motion Range and Heavy Duty 2-DOF Spherical Parallel Wrist Mechanism,” J. Robot. Mechatron., Vol.25, No.2, pp. 294-305, 2013. https://doi.org/10.20965/jrm.2013.p0294
- [2] K. Miyata, T. Sasagawa, T. Doi, and K. Tadakuma, “A Study of Leg-Type Landing Gear for Aerial Vehicles – Development of One Leg Model –,” J. Robot. Mechatron., Vol.23, No.2, pp. 266-270, 2011. https://doi.org/10.20965/jrm.2011.p0266
- [3] H. Kino and S. Kawamura, “Mechanism and Control of Parallel-Wire Driven System,” J. Robot. Mechatron., Vol.27, No.6, pp. 599-607, 2015. https://doi.org/10.20965/jrm.2015.p0599
- [4] K. Misu, M. Ikeda, K. Or, M. Ando, M. Gunji, H. Mochiyama, and R. Niiyama, “Robostrich Arm: Wire-Driven High-DOF Underactuated Manipulator,” J. Robot. Mechatron., Vol.34, No.2, pp. 328-338, 2022. https://doi.org/10.20965/jrm.2022.p0328
- [5] G. Endo, A. Horigome, and A. Takata, “Super Dragon: A 10-m-Long-Coupled Tendon-Driven Articulated Manipulator,” IEEE Robot. Autom. Lett., Vol.4, No.2, pp. 934-941, 2019. https://doi.org/10.1109/LRA.2019.2894855
- [6] A. Fukuhara, M. Gunji, Y. Masuda, K. Tadakuma, and A. Ishiguro, “Flexible Shoulder in Quadruped Animals and Robots Guiding Science of Soft Robotics,” J. Robot. Mechatron., Vol.34, No.2, pp. 304-309, 2022. https://doi.org/10.20965/jrm.2022.p0304
- [7] D. Huczala, T. Kot, M. Pfurner, V. Krys, and Z. Bobovský, “Multirepresentations and Multiconstraints Approach to the Numerical Synthesis of Serial Kinematic Structures of Manipulators,” IEEE Access, Vol.10, pp. 68937-68951, 2022. https://doi.org/10.1109/ACCESS.2022.3186098
- [8] M. A. Laribi, L. Romdhane, and S. Zeghloul, “Analysis and Optimal Synthesis of Single-Loop Spatial Mechanisms,” J. Zhejiang Univ. Sci., Vol.A12, pp. 665-679, 2011. https://doi.org/10.1631/jzus.A1000052
- [9] S. Ha, S. Coros, A. Alspach, J. M. Bern, J. Kim, and K. Yamane, “Computational Design of Robotic Devices From High-Level Motion Specifications,” IEEE Trans. Robot., Vol.34, No.5, pp. 1240-1251, 2018. https://doi.org/10.1109/TRO.2018.2830419
- [10] R. Koike, R. Ariizumi, and F. Matsuno, “Automatic Robot Design Inspired by Evolution of Vertebrates,” Artif. Life Robot., Vol.27, pp. 624-631, 2022. https://doi.org/10.1007/s10015-022-00793-4
- [11] J. Whitman and H. Choset, “Task-Specific Manipulator Design and Trajectory Synthesis,” IEEE Robot. Autom. Lett., Vol.4, No.2, pp. 301-308, 2019. https://doi.org/10.1109/LRA.2018.2890206
- [12] S. Ha, S. Coros, A. Alspach, J. Kim, and K. Yamane, “Computational Co-Optimization of Design Parameters and Motion Trajectories for Robotic Systems,” Int. J. Rob. Res., Vol.37, Nos.13-14, pp. 1521-1536, 2018. https://doi.org/10.1177/0278364918771172
- [13] S. Shirafuji and J. Ota, “Kinematic Synthesis of a Serial Robotic Manipulator by Using Generalized Differential Inverse Kinematics,” IEEE Trans. Robot., Vol.35, No.4, pp. 1047-1054, 2019. https://doi.org/10.1109/TRO.2019.2907810
- [14] T. Harada and J. Angeles, “From the McGill Pepper-Mill Carrier to the Kindai ATARIGI Carrier: A Novel Two Limbs Six-DOF Parallel Robot With Kinematic and Actuation Redundancy,” IEEE Int. Conf. Robot. Autom. (ROBIO), pp. 1328-1333, 2017. https://doi.org/10.1109/ROBIO.2017.8324601
- [15] C.-C. Lee and J. M. Hervé, “New 6-Screw Linkage With Circular Translation and its Variants,” Mech. Mach. Theory, Vol.85, pp. 205-219, 2015. https://doi.org/10.1016/j.mechmachtheory.2014.11.018
- [16] N. Kimura and N. Iwatsuki, “A Semi-Automatic Type Synthesis of a Closed-Loop Spatial Path-Generator,” G. Venture, J. Solis, Y. Takeda, and A. Konno (Eds.) “ROMANSY 23 – Robot Design, Dynamics and Control,” CISM Int. Centre for Mechanical Sciences, Vol.601, Springer Cham, 2020. https://doi.org/10.1007/978-3-030-58380-4_31
- [17] K. M. Lynch and F. C. Park, “Modern Robotics: Mechanics, Planning, and Control (1st. ed.),” Cambridge University Press, 2017.
- [18] A. Perez-Gracia and J. M. McCarthy, “Kinematic Synthesis of Spatial Serial Chains Using Clifford Algebra Exponentials,” Proc. Inst. Mech. Eng., Part C: J. Mech. Eng. Sci., Vol.220, No.7, pp. 953-968, 2006. https://doi.org/10.1243/09544062JMES166
- [19] L. J. Eshleman and J. D. Schaffer, “Real-Coded Genetic Algorithms and Interval-Schemata: Foundations of Genetic Algorithms,” Elsevier, Vol.2, pp. 187-202, 1993.
This article is published under a Creative Commons Attribution-NoDerivatives 4.0 Internationa License.