Paper:
Fabrication of a Magnetically Driven Cell-Stretching Device for Predefined Cell Alignment in Vitro
Tasuku Nakahara
, Sora Ono, and Kazuyuki Minami
Graduate School of Sciences and Technology for Innovation, Yamaguchi University
2-16-1 Tokiwadai, Ube, Yamaguchi 755-8611, Japan
Various devices have been developed that use stretching silicone sheets to evaluate cellular mechanotransduction. However, few studies have explored predefined cell alignments using mechanical stimuli for engineering applications, including cell sheets and drug screenings. Therefore, we proposed a magnetically driven cell-stretching device for predefined cell alignment in vitro, which consisted mainly of a circular silicone membrane with a neodymium magnet and standard cell culture dish. As the proposed device was incorporated into a cell culture dish, there may be a small risk of contamination in long-term incubation experiments. The device was fabricated by assembling a polydimethylsiloxane membrane and silicone ring. The fabricated device showed that the membrane strain increased with increasing voltage application to the electromagnet, and indicated that cell alignment occurs when strain exceeds 0.8%. Following cyclic stimulation of cells adhered to a membrane for 4 h in a CO2 incubator with 1.05% strain at 0.1 Hz, cell alignment with the predefined direction increased by 20.4% compared to that before stimulation. The findings imply that the proposed device may be utilized for predefined cell alignment.
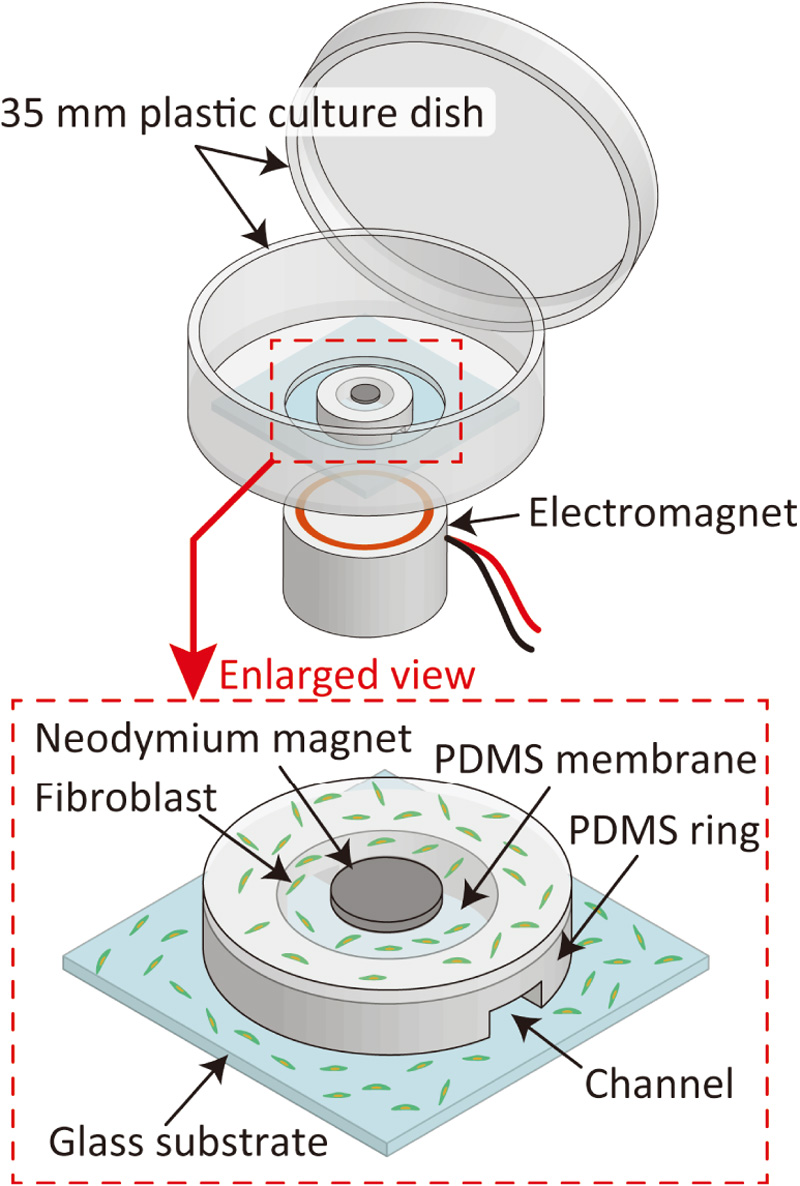
Magnetically driven cell-stretching device
- [1] F. Boccafoschi, M. Bosetti et al., “Dynamic Fibroblast Cultures,” Cell Adhesion & Migration, Vol.1, No.3, pp. 124-128, 2007. https://doi.org/10.4161/cam.1.3.5144
- [2] H. Gao, M. Zhai et al., “Low-level mechanical vibration enhances osteoblastogenesis via a canonical Wnt signaling-associated mechanism,” Mol. Med. Rep., Vol.16, No.1, pp. 317-324, 2017. https://doi.org/http://dx.doi.org/10.3892/mmr.2017.6608
- [3] K. Sato and D. Oomori, “Development of vibration mechanical stimuli loading device for live cell fluorescence microscopy,” J. Biomech. Sci. Eng., Vol.17, No.2, Article No.21-00294, 2022. https://doi.org/10.1299/jbse.21-00294
- [4] S. Giulitti, A. Zambon et al., “Mechanotransduction through substrates engineering and microfluidic devices,” Current Opinion in Chemical Engineering, Vol.11, pp. 67-76, 2016. https://doi.org/10.1016/j.coche.2016.01.010
- [5] S. Higgins, J. S. Lee et al., “Inducing neurite outgrowth by mechanical cell stretch,” Biores Open Access, Vol.2, No.3, pp. 212-216, 2013. https://doi.org/10.1089/biores.2013.0008
- [6] W. Huang, S. Zhang et al., “Three-Motorized-Stage Cyclic Stretching System for Cell Monitoring Based on Chamber Local Displacement Waveforms,” Applied Sciences, Vol.9, No.8, Article No.1560, 2019. https://doi.org/10.3390/app9081560
- [7] A. Matsugaki, N. Fujiwara et al., “Continuous cyclic stretch induces osteoblast alignment and formation of anisotropic collagen fiber matrix,” Acta Biomater., Vol.9, No.7, pp. 7227-7235, 2013. https://doi.org/10.1016/j.actbio.2013.03.015
- [8] J. Kreutzer, M. Viehrig et al., “Pneumatic unidirectional cell stretching device for mechanobiological studies of cardiomyocytes,” Biomech. Model. Mechanobiol., Vol.19, No.1, pp. 291-303, 2020. https://doi.org/10.1007/s10237-019-01211-8
- [9] H. Kamble, R. Vadivelu et al., “An Electromagnetically Actuated Double-Sided Cell-Stretching Device for Mechanobiology Research,” Micromachines (Basel), Vol.8, No.8, Article No.256, 2017. https://doi.org/10.3390/mi8080256
- [10] D. Huh, B. D. Matthews et al., “Reconstituting organ-level lung functions on a chip,” Science, Vol.328, No.5986, pp. 1662-1668, 2010. https://doi.org/10.1126/science.1188302
- [11] L. Huang, P. S. Mathieu et al., “A stretching device for high-resolution live-cell imaging,” Ann. Biomed. Eng., Vol.38, No.5, pp. 1728-1740, 2010. https://doi.org/10.1007/s10439-010-9968-7
- [12] G. Subramanian, M. Elsaadany et al., “Creating homogenous strain distribution within 3D cell-encapsulated constructs using a simple and cost-effective uniaxial tensile bioreactor: Design and validation study,” Biotechnol. Bioeng., Vol.114, No.8, pp. 1878-1887, 2017. https://doi.org/10.1002/bit.26304
- [13] J. Kreutzer, L. Ikonen et al., “Pneumatic cell stretching system for cardiac differentiation and culture,” Med. Eng. Phys., Vol.36, No.4, pp. 496-501, 2014. https://doi.org/10.1016/j.medengphy.2013.09.008
- [14] S. Kollimada, S. Balakrishnan et al., “A micro-mechanical device for in-situ stretching of single cells cultured on it,” J. of Micro-Bio Robotics, Vol.13, No.1, pp. 27-37, 2017. https://doi.org/10.1007/s12213-017-0102-x
- [15] D. B. Serrell, J. Law et al., “A uniaxial bioMEMS device for imaging single cell response during quantitative force-displacement measurements,” Biomed. Microdevices, Vol.10, No.6, Article No.883, 2008. https://doi.org/10.1007/s10544-008-9202-7
- [16] K. Minami, T. Hayashi et al., “Development of micro mechanical device having two-dimensional array of micro chambers for cell stretching,” Biomed. Microdevices, Vol.20, No.1, Article No.10, 2018. https://doi.org/10.1007/s10544-017-0256-2
- [17] K. Mineda, K. Sato et al., “Cyclical Stretching Induces Excess Intracellular Ca2+ Influx in Human Keloid-Derived Fibroblasts In Vitro,” Plast. Reconstr. Surg., Vol.151, No.2, pp. 346-354, 2023. https://doi.org/10.1097/PRS.0000000000009843
- [18] H. Kamble, R. Vadivelu et al., “Pneumatically actuated cell-stretching array platform for engineering cell patterns in vitro,” Lab Chip, Vol.18, No.5, pp. 765-774, 2018. https://doi.org/10.1039/C7LC01316G
- [19] G. Wagner, L. Sievers et al., “Impact of Immunosuppressive Drugs on Fibroblasts: An In Vitro Study,” J. Clin. Med., Vol.11, No.11, Article No.3107, 2022. https://doi.org/10.3390/jcm11113107
- [20] S. Chagnon-Lessard, H. Jean-Ruel et al., “Cellular orientation is guided by strain gradients,” Integr. Biol., Vol.9, No.7, pp. 607-618, 2017. https://doi.org/10.1039/C7IB00019G
- [21] S. Bhattacharya, A. Datta et al., “Studies on surface wettability of poly(dimethyl) siloxane (PDMS) and glass under oxygen-plasma treatment and correlation with bond strength,” J. Microelectromech Sys., Vol.14, No.3, pp. 590-597, 2005. https://doi.org/10.1109/JMEMS.2005.844746
- [22] C. C. Shih, S. J. Lin et al., “The cytotoxicity of corrosion products of nitinol stent wire on cultured smooth muscle cells,” J. Biomed. Mater. Res., Vol.52, No.2, pp. 395-403, 2000. https://doi.org/10.1002/1097-4636(200011)52:2<395::aid-jbm21>3.0.co;2-b
- [23] R. Rezakhaniha, A. Agianniotis et al., “Experimental investigation of collagen waviness and orientation in the arterial adventitia using confocal laser scanning microscopy,” Biomech. Model. Mechanobiol., Vol.11, Nos.3-4, pp. 461-473, 2012. https://doi.org/10.1007/s10237-011-0325-z
- [24] T. Ota, M. Chiba et al., “Vibrational stimulation induces osteoblast differentiation and the upregulation of osteogenic gene expression in vitro,” Cytotechnology, Vol.68, No.6, pp. 2287-2299, 2016. https://doi.org/10.1007/s10616-016-0023-x
- [25] J. Miyakoshi, “Effects of static magnetic fields at the cellular level,” Prog. Biophys. Mol. Biol., Vol.87, Nos.2-3, pp. 213-223, 2005. https://doi.org/10.1016/j.pbiomolbio.2004.08.008
- [26] M. Iwasaka, J. Miyakoshi et al., “Magnetic Field Effects on Assembly Pattern of Smooth Muscle Cells,” In Vitro Cellular & Developmental Biology – Animal, Vol.39, Nos.3-4, pp. 120-123, 2003. https://doi.org/10.1007/s11626-003-0005-0
- [27] L. Wang, Y. Li et al., “Patterning Cellular Alignment through Stretching Hydrogels with Programmable Strain Gradients,” ACS Applied Materials & Interfaces, Vol.7, No.27, pp. 15088-15097, 2015. https://doi.org/10.1021/acsami.5b04450
This article is published under a Creative Commons Attribution-NoDerivatives 4.0 Internationa License.