Paper:
Characteristics of Pneumatic Artificial Rubber Muscle Using Two Shape-Memory Polymer Sheets
Kazuto Takashima*, Daiki Iwamoto*, Shun Oshiro*, Toshiro Noritsugu**, and Toshiharu Mukai***
*Graduate School of Life Science and Systems Engineering, Kyushu Institute of Technology
2-4 Hibikino, Wakamatsu-ku, Kitakyushu, Fukuoka 808-0196, Japan
**Mimasaka University
50 Kitazonocho, Tsuyama, Okayama 708-8511, Japan
***Department of Information Engineering, Faculty of Science and Technology, Meijo University
1-501 Shiogamaguchi, Tenpaku-ku, Nagoya, Aichi 468-8502, Japan
We have developed a pneumatic artificial rubber muscle having a bending direction that can be changed using two shape-memory polymer (SMP) sheets, the stiffness of which depends on the temperature. In the present study, we attached two SMP sheets with embedded electrical heating wires to both sides of a pneumatic artificial rubber muscle in order to realize multidirectional actuation and evaluated the basic characteristics of the artificial muscle. The actuator is based on the design of a conventional curved-type artificial rubber muscle. Since only a heated SMP sheet becomes soft, the rigid SMP sheet inhibits the extension of the side of the actuator. Therefore, bending motion can be induced when air is supplied to the internal bladder. By controlling the temperature of the SMP sheets, the bending direction of the prototype actuator could be changed. Namely, three kinds of motions, such as two-directional bending and axial extension, became possible. Moreover, we improved the manufacturing method and the structure of the artificial muscle, such as the stitching method and the SMP sheet thickness, and evaluated the characteristics of the two-directional bending and the axial extension motions of the prototype actuator. We also calculated the theoretical values and compared these values with the experimental results. Furthermore, we examined the application of the actuators to a robot hand. Using the two-directional motion of the actuator, the proposed robot hand can grasp either small or large objects. The experimental results conducted using this prototype confirm the feasibility of the newly proposed actuator.
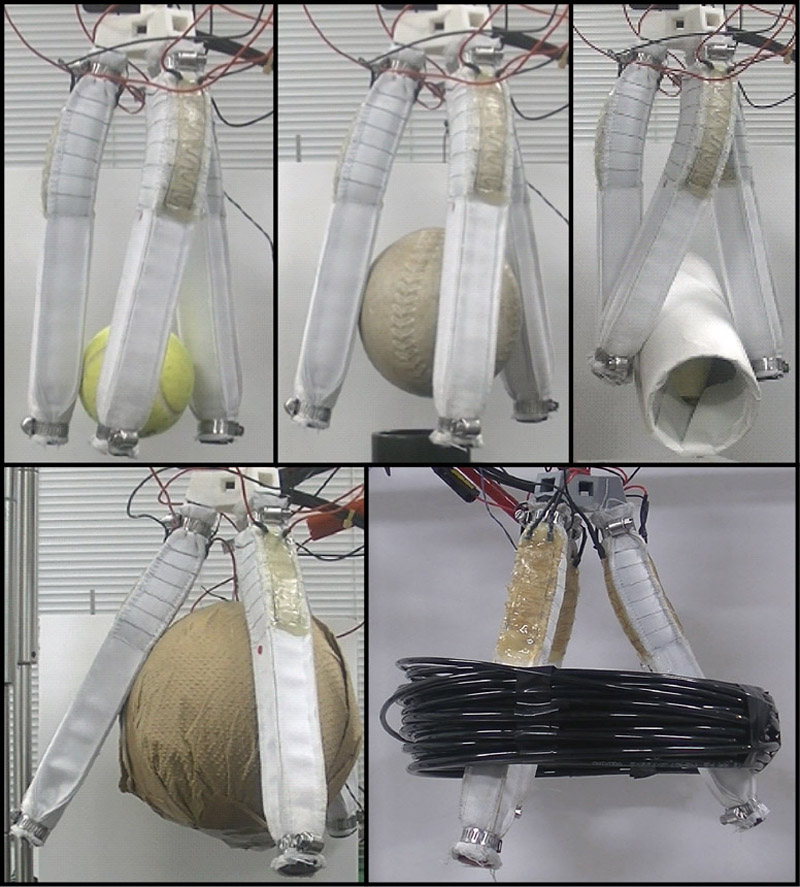
Robot hand consisting of artificial muscles
- [1] T. Higuchi, K. Suzumori, and S. Tadokoro (Eds.), “Next-generation actuators leading breakthroughs,” Springer, 2010.
- [2] M. Aragane, T. Noritsugu, M. Takaiwa, D. Sasaki, and S. Naomoto, “Development of sheet-like curved type pneumatic rubber muscle and application to elbow power assist wear,” J. Robotics Society of Japan, Vol.26, No.6, pp. 674-682, doi: 10.7210/jrsj.26.674, 2008 (in Japanese).
- [3] J. Shintake, V. Cacucciolo, D. Floreano, and H. Shea, “Soft robotic grippers,” Adv. Mater., Vol.30, 1707035, doi: 10.1002/adma.201707035, 2018.
- [4] S. Yahara, S. Wakimoto, T. Kanda, and K. Matsushita, “McKibben artificial muscle realizing variable contraction characteristics using helical shape-memory polymer fibers,” Sensors and Actuators: A Physical, Vol.295, pp. 637-642, doi: 10.1016/j.sna.2019.06.012, 2019.
- [5] K. Suzumori, S. Iikura, and H. Tanaka, “Applying a flexible microactuator to robotic mechanisms,” IEEE Control Systems Magazine, Vol.12, pp. 21-27, doi: 10.1109/37.120448, 1992.
- [6] K. Suzumori, “New robotics pioneered by fluid power,” J. Robot. Mechatron., Vol.32, No.5, pp. 854-862, doi: 10.20965/jrm.2020.p0854, 2020.
- [7] T. Vo-Minh, T. Tjahjowidodo, H. Ramon, and H. Van Brussel, “A new approach to modeling hysteresis in a pneumatic artificial muscle using the Maxwell-slip model,” IEEE ASME Trans. Mechatron., Vol.16, No.1, pp. 177-186, doi: 10.1109/TMECH.2009.2038373, 2011.
- [8] R. Deimel and O. Brock, “A novel type of compliant and underactuated robotic hand for dexterous grasping,” The Int. J. of Robotics Res., Vol.35, pp. 161-185, doi: 10.1177/0278364915592961, 2016.
- [9] K. Hosoda, T. Takuma, A. Nakamoto, and S. Hayashi, “Biped robot design powered by antagonistic pneumatic actuators for multi-modal locomotion,” Robotics and Autonomous Systems, Vol.56, pp. 46-53, doi: 10.1016/j.robot.2007.09.010, 2008.
- [10] F. Ilievski, A. D. Mazzeo, R. F. Shepherd, X. Chen, and G. M. Whitesides, “Soft robotics for chemists,” Angew. Chemie – Int. Ed., Vol.50, No.8, pp. 1890-1895, doi: 10.1002/anie.201006464, 2011.
- [11] H. Zhao, K. O’Brien, S. Li, and R. F. Shepherd, “Optoelectronically innervated soft prosthetic hand via stretchable optical waveguides,” Science Robotics, Vol.1, eaai7529, doi: 10.1126/scirobotics.aai7529, 2016.
- [12] Z. Wang, M. Zhu, S. Kawamura, and S. Hirai, “Fabrication and performance comparison of different soft pneumatic actuators for lunch box packaging,” Proc. of IEEE Int. Conf. on Real-Time Computing and Robotics (RCAR) Vol.2017-July, pp. 22-27, doi: 10.1109/RCAR.2017.8311830, 2017.
- [13] W. Zhou and Y. Li, “Modeling and analysis of soft pneumatic actuator with symmetrical chambers used for bionic robotic fish,” Soft Robot, Vol.7, No.2, pp. 168-178, doi: 10.1089/soro.2018.0087, 2020.
- [14] K. Nishikawa, K. Hirata, and M. Takaiwa, “Development of pneumatically driven hand capable of grasping flexible objects,” J. Robot. Mechatron., Vol.32, No.5, pp. 923-930, doi: 10.20965/jrm.2020.p0923, 2020.
- [15] W. Tian, C. Jhan, M. Inokuma, T. Akagi, S. Dohta, and S. Shimooka, “Development of a tetrahedral-shaped soft robot arm as a wrist rehabilitation device using extension type flexible pneumatic actuators,” J. Robot. Mechatron., Vol.32, No.5, pp. 931-938, doi: 10.20965/jrm.2020.p0931, 2020.
- [16] J. Tanaka and N. Matsuhira, “Disposable robotic finger driven pneumatically by flat tubes and a hollow link mechanism,” J. Robot. Mechatron., Vol.32, No.5, pp. 958-976, doi: 10.20965/jrm.2020.p0958, 2020.
- [17] K. Takashima, J. Rossiter, and T. Mukai, “McKibben artificial muscle using shape-memory polymer,” Sensors and Actuators: A Physical, Vol.164, pp. 116-124, doi: 10.1016/j.sna.2010.09.010, 2010.
- [18] K. Takashima, T. Noritsugu, J. Rossiter, S. Guo, and T. Mukai, “Curved type pneumatic artificial rubber muscle using shape-memory polymer,” J. Robot. Mechatron., Vol.24, No.3, pp. 472-479, doi: 10.20965/jrm.2012.p0472, 2012.
- [19] K. Takashima, K. Sugitani, N. Morimoto, S. Sakaguchi, T. Noritsugu, and T. Mukai, “Pneumatic artificial rubber muscle using shape-memory polymer sheet with embedded electrical heating wire,” Smart Mater. Struct., Vol.23, No.12, 125005, doi: 10.1088/0964-1726/23/12/125005, 2014.
- [20] K. Takashima, H. Kamizono, M. Takenaka, and T. Mukai, “Force sensor utilizing stiffness change of shape-memory polymer based on temperature,” ROBOMECH J., Vol.4, 17, doi: 10.1186/s40648-017-0086-2, 2017.
- [21] H. Tobushi, K. Okumura, S. Hayashi, and N. Ito, “Thermomechanical constitutive model of shape memory polymer,” Mech. Mater., Vol.33, pp. 545-554, doi: 10.1016/S0167-6636(01)00075-8, 2001.
- [22] H. Tobushi, H. Hara, E. Yamada, and S. Hayashi, “Thermomechanical properties in a thin film of shape memory polymer of polyurethane series,” Smart Mater. Struct., Vol.5, pp. 483-491, doi: 10.1088/0964-1726/5/4/012, 1996.
- [23] H. Meng, H. Mohamadian, M. Stubblefield, D. Jerro, S. Ibekwe, S.-S. Pang, and G. Li, “Various shape memory effects of stimuli-responsive shape memory polymers,” Smart Mater. Struct., Vol.22, No.9, 093001, doi: 10.1088/0964-1726/22/9/093001, 2013.
- [24] M. Behl and A. Lendlein, “Shape-memory polymers,” Mater. Today, Vol.10, No.4, pp. 20-28, doi: 10.1016/S1369-7021(07)70047-0, 2007.
- [25] Q. Ge, C. K. Dunn, H. J. Qi, and M. L. Dunn, “Active origami by 4D printing,” Smart Mater. Struct., Vol.23, No.9, 094007, doi: 10.1088/0964-1726/23/9/094007, 2014.
- [26] Y. Yang, Y. Chen, Y. Li, and M. Z. Chen, “3D printing of variable stiffness hyper-redundant robotic arm,” Proc. of IEEE Int. Conf. on Robotics and Automation (ICRA), Vol.2016-June, pp. 3871-3877, doi: 10.1109/ICRA.2016.7487575, 2016.
- [27] Y. An and H. Okuzaki, “Novel electro-active shape memory polymers for soft actuators,” Jpn. J. Appl. Phys., Vol.59, 061002, doi: 10.35848/1347-4065/ab8e08, 2020.
This article is published under a Creative Commons Attribution-NoDerivatives 4.0 Internationa License.