Paper:
Application of Inertial and GNSS Integrated Navigation to Seabird Biologging
Masaru Naruoka*1, Yusuke Goto*2, Henri Weimerskirch*2, Takashi Mukai*3, Taichi Sakamoto*3, Kentaro Q. Sakamoto*4, and Katsufumi Sato*4
*1Aeronautical Technology Directorate, Japan Aerospace Exploration Agency (JAXA)
6-13-1 Osawa, Mitaka-shi, Tokyo 181-0015, Japan
*2Centre d’Etudes Biologiques Chizé (CNRS)
79360 Villiers-en-Bois, France
*3ATTACCATO Limited Liability Company
Osaka Research Institute of Industrial Science and Technology Izumi Center F104, 2-7-1 Ayumino, Izumi-shi, Osaka 594-1157, Japan
*4Department of Marine Bioscience, Atmosphere and Ocean Research Institute, The University of Tokyo
5-1-5 Kashiwanoha, Kashiwa, Chiba 277-8564, Japan
The study demonstrates the versatility of integration of inertial navigation and global navigation satellite system (GNSS) with its unique application to seabird biologging. Integrated navigation was originally developed in the field of aerospace engineering, which requires accurate and reliable position, velocity, and attitude information for the guidance and control of aircraft and spacecraft. Due to its high performance and recent progress of sensor development, integrated navigation has been widely used not only in aerospace but also in many fields represented by land and marine vehicles. One of its ultimate applications under the constraint on the size and power consumption of devices is this study. Seabird biologging involves attaching a logging device onto a seabird for scientific purposes to understand its biomechanics, behavior, and so on. Design restrictions for the device include several tens of grams mass, several tens of millimeters in length, and several tens of milliamperes of power consumption. It is more difficult to maintain the accuracy of such a device than applications to an artificial vehicle. This study has shown that integrated navigation is a feasible solution for such extreme applications with two examples: biologging for wandering albatrosses and great frigatebirds. Furthermore, it should be stressed that the navigation captured the world’s first data of their detailed trajectories and attitudes in their dynamic and thermal soarings. For completeness, the navigation algorithm, simulation results to show the effectiveness of the algorithm, and the logging devices attached to bird are also described.
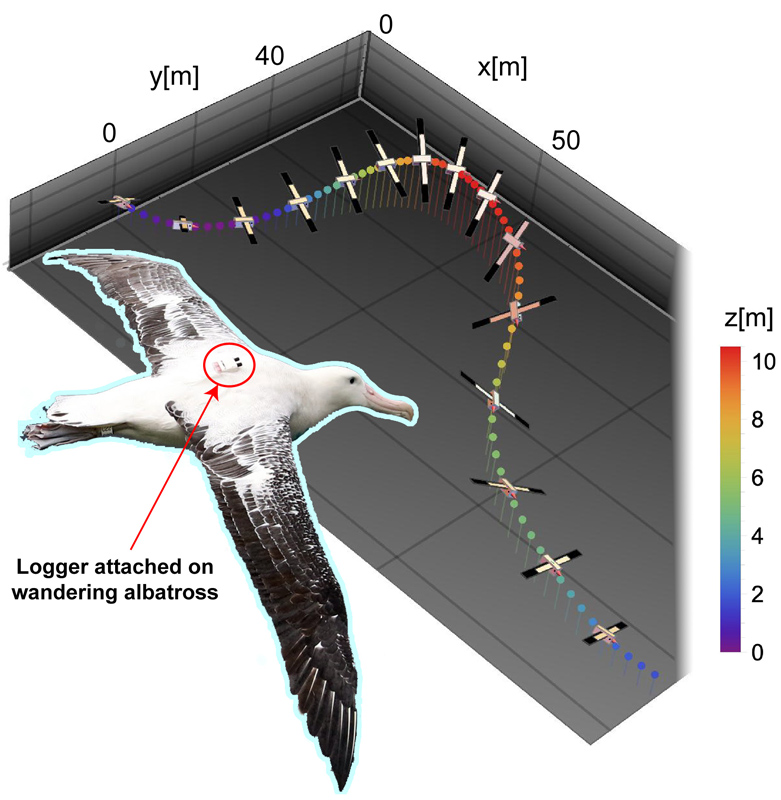
Dynamic soaring measured by inertial/GNSS
- [1] R. Kays et al., “Terrestrial animal tracking as an eye on life and planet,” Science, Vol.348, Issue 6240, aaa2478, 2015.
- [2] N. E. Hussey et al., “Aquatic animal telemetry: a panoramic window into the underwater world,” Science, Vol.348, Issue 6240, 1255642, 2015.
- [3] N. L. Payne et al., “From physiology to physics: are we recognizing the flexibility of biologging tools?,” J. of Experimental Biology, Vol.217, Issue 3, pp. 317-322, 2014.
- [4] K. Sato et al., “Scaling of soaring seabirds and implications for flight abilities of giant pterosaurs,” PLOS ONE, Vol.4, No.4, e5400, 2009.
- [5] K. Sato et al., “Scaling of swim speed and stroke frequency in geometrically similar penguins: they swim optimally to minimize cost of transport,” Proc. of the Royal Society B, Biological Sciences, Vol.277, Issue 1682, pp. 707-714, 2010.
- [6] Y. Y. Watanabe et al., “Scaling of swim speed in breath-hold divers,” J. of Animal Ecology, Vol.80, Issue 1, pp. 57-68, 2011.
- [7] G. Sachs et al., “Experimental verification of dynamic soaring in albatrosses,” J. of Experimental Biology, Vol.216, Issue 22, pp. 4222-4232, 2013.
- [8] H. J. Williams et al., “Vultures respond to challenges of near-ground thermal soaring by varying bank angle,” J. of Experimental Biology, Vol.221, Issue 23, jeb174995, 2018.
- [9] G. Sachs, “Minimum shear wind strength required for dynamic soaring of albatrosses,” Int. J. of Avian Science, Vol.147, Issue 1, pp. 1-10, 2005.
- [10] G. D. Bousquet, M. S. Triantafyllou, and J.-J. E. Slotine, “Optimal dynamic soaring consists of successive shallow arcs,” J. of The Royal Society Interface, Vol.14, Issue 135, 20170496, 2017.
- [11] C. J. Pennycuick, “Thermal soaring compared in three dissimilar tropical bird species, Fregata magnificens, Pelecanus occidentals and Coragyps atratus,” J. of Experimental Biology, Vol.102, Issue 1, pp. 307-325, 1983.
- [12] G. Reddy et al., “Learning to soar in turbulent environments,” Proc. of the National Academy of Sciences, Vol.113, No.33, pp. E4877-E4884, 2016.
- [13] G. Reddy et al., “Glider soaring via reinforcement learning in the field,” Nature, Vol.562, Issue 7726, pp. 236-239, 2018.
- [14] K. Shiomi et al., “Effect of ocean current on the dead-reckoning estimation of 3-D dive paths of emperor penguins,” Aquatic Biology, Vol.3, No.3, pp. 265-270, 2008.
- [15] T. Noda et al., “Animal-mounted gyroscope/accelerometer/ magnetometer: In situ measurement of the movement performance of fast-start behaviour in fish,” J. of Experimental Marine Biology and Ecology, Vol.451, pp. 55-68, 2014.
- [16] S. O. H. Madgwick, A. J. L. Harrison, and R. Vaidyanathan, “Estimation of IMU and MARG orientation using a gradient descent algorithm,” 2011 IEEE Int. Conf. on Rehabilitation Robotics, pp. 1-7, 2011.
- [17] R. M. Rogers, “Applied Mathematics in Integrated Navigation Systems, Third Edition,” American Institute of Aeronautics and Astronautics, 2007.
- [18] W. Li and J. Wang, “Effective Adaptive Kalman Filter for MEMS-IMU/Magnetometers Integrated Attitude and Heading Reference Systems,” J. of Navigation, Vol.66, Issue 1, pp. 99-113, 2013.
- [19] J. Wang et al., “Integration of GPS/INS/vision sensors to navigate unmanned aerial vehicles,” The Int. Archives of the Photogrammetry, Remote Sensing and Spatial Information Sciences, Vol.37, part B1, pp. 963-969, 2008.
- [20] D. A. Anderson and J. H. Doty, “GPS-IMU-Loran Integration for Airborne Applications,” Proc. of the 2004 National Technical Meeting of the Institute of Navigation, pp. 1077-1086, 2004.
- [21] N. Barbour and G. Schmidt, “Inertial sensor technology trends,” IEEE Sensors J., Vol.1, No.4, pp. 332-339, 2001.
- [22] V. Passaro et al., “Gyroscope technology and applications: A review in the industrial perspective,” Sensors, Vol.17, Issue 10, 2284, 2017.
- [23] M. Naruoka and T. Tsuchiya, “High-Performance Navigation System with Integration of Low-Precision MEMS INS and General-Purpose GPS,” Trans. of the Japan Society for Aeronautical and Space Sciences, Vol.50, Issue 170, pp. 284-292, 2008.
- [24] G. Falco, M. Pini, and G. Marucco, “Loose and Tight GNSS/INS Integrations: Comparison of Performance Assessed in Real Urban Scenarios,” Sensors, Vol.17, Issue 2, 255, 2017.
- [25] G. Gao and G. Lachapelle, “A novel architecture for ultra-tight HSGPS-INS integration,” J. of Global Positioning Systems, Vol.7, Issue 1, pp. 46-61, 2008.
- [26] S. H. Oh and D.-H. Hwang, “Low-cost and high performance ultra-tightly coupled GPS/INS integrated navigation method,” Advances in Space Research, Vol.60, Issue 12, pp. 2691-2706, 2017.
- [27] GPS ICD, “Global positioning systems directorate system engineering & integration interface specification IS-GPS-200H,” Navstar GPS Space Segment/Navigation User Interfaces, 2013.
- [28] U.S. Department of Defense, “Global Positioning System Standard Positioning Service (SPS) Performance Standard, 5th Edition,” Technical Report, 2020.
- [29] E. Thébault et al., “International geomagnetic reference field: the 12th generation,” Earth, Planets and Space, Vol.67, No.1, 79, 2015.
- [30] ISO 2533:1975, “Standard Atmosphere,” Standard, Int. Organization for Standardization, 1975.
- [31] National Imagery and Mapping Agency, “Department of Defense World Geodetic System 1984: its definition and relationships with local geodetic systems,” Technical Report TR8350.2, 2000.
- [32] T. Mukai et al., “Cycle Performance of Silicon Electrode Coated with Silicate Based Inorganic Binder for High Energy Density Li-ion Secondary Battery,” Function and Materials, Vol.38, No.11, pp. 19-24, 2018 (in Japanese).
- [33] T. Sakamoto et al., “NCA/Si Cell Development and Biologging Demonstration Test,” The 60th Battery Symp. in Japan, 2B25, 2019 (in Japanese).
- [34] S. P. Vandenabeele et al., “When three per cent may not be three per cent; device-equipped seabirds experience variable flight constraints,” Marine Biology, Vol.159, Issue 1, pp. 1-14, 2011.
- [35] K. Sato, “Factors affecting stroking patterns and body angle in diving Weddell seals under natural conditions,” J. of Experimental Biology, Vol.206, Issue 9, pp. 1461-1470, 2003.
- [36] S. Kawatsu et al., “A New Method to Calibrate Attachment Angles of Data Loggers in Swimming Sharks,” EURASIP J. on Advances in Signal Processing, Vol.2010, No.1, 732586, 2009.
- [37] H. Weimerskirch et al., “Lifetime foraging patterns of the wandering albatross: life on the move!,” J. of Experimental Marine Biology and Ecology, Vol.450, pp. 68-78, 2014.
- [38] H. Weimerskirch et al., “Changes in wind pattern alter albatross distribution and life-history traits,” Science, Vol.335, Issue 6065, pp. 211-214, 2012.
- [39] A. Corbeau et al., “How do seabirds modify their search behaviour when encountering fishing boats?,” PLOS ONE, Vol.14, No.9, e0222615, 2019.
- [40] H. Weimerskirch et al., “Frigate birds track atmospheric conditions over months-long transoceanic flights,” Science, Vol.353, Issue 6294, pp. 74-78, 2016.
- [41] H. Takahashi et al., “Seabird Biologging System with Compact Waterproof Airflow Sensor,” J. Robot. Mechatron., Vol.33, No.3, pp. 466-474, 2021.
This article is published under a Creative Commons Attribution-NoDerivatives 4.0 Internationa License.