Paper:
The Understanding of ON-Edge Motion Detection Through the Simulation Based on the Connectome of Drosophila’s Optic Lobe
Munehiro Hayashi*, Tomoki Kazawa**, Hayato Tsunoda***, and Ryohei Kanzaki**
*Graduate School of Engineering, The University of Tokyo
4-6-1 Komaba, Meguro-ku, Tokyo 153-8904, Japan
**Research Center for Advanced Science and Technology, The University of Tokyo
4-6-1 Komaba, Meguro-ku, Tokyo 153-8904, Japan
***Department of Mechano-Informatics, Graduate School of Information Science and Technology, The University of Tokyo
4-6-1 Komaba, Meguro-ku, Tokyo 153-8904, Japan
The optic lobe of the fly is one of the prominent model systems for the neural mechanism of the motion detection. How a fly who lives under various visual situations of the nature processes the information from at most a few thousands of ommatidia in their neural circuit for the detection of moving objects is not exactly clear though many computational models of the fly optic lobe as a moving objects detector were suggested. Here we attempted to elucidate the mechanisms of ON-edge motion detection by a simulation approach based on the TEM connectome of Drosophila. Our simulation model of the optic lobe with the NEURON simulator that covers the full scale of ommatidia, reproduced the characteristics of the receptor neurons, lamina monopolar neurons, and T4 cells in the lobula. The contribution of each neuron can be estimated by changing synaptic connection strengths in the simulation and measuring the response to the motion stimulus. Those show the paradelle pathway provide motion detection in the fly optic lobe has more robustness and is more sophisticated than a simple combination of HR and BL systems.
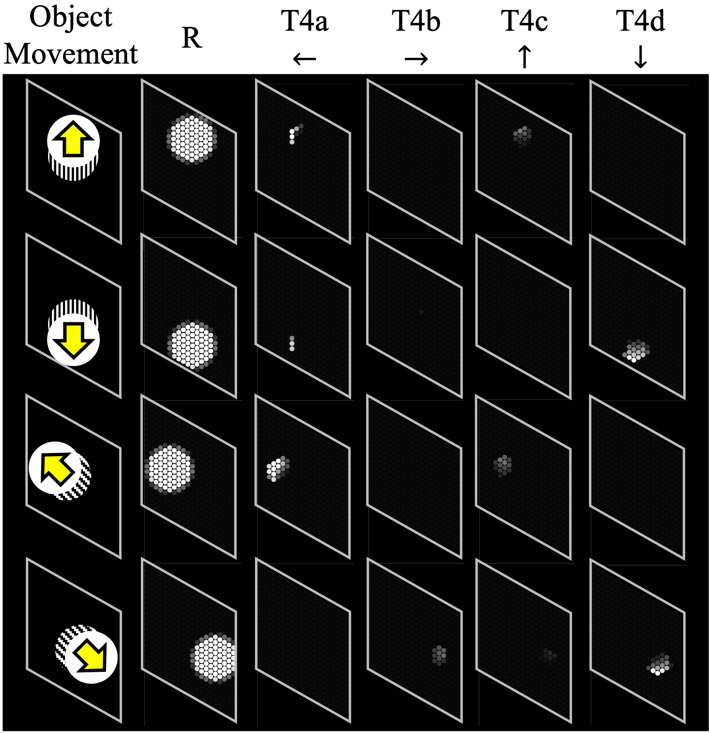
ON-edge detection to the object movement
- [1] F. T. Muijres, M. J. Elzinga, J. M. Melis, and M. H. Dickinson, “Flies evade looming targets by executing rapid visually directed banked turns,” Science, Vol.344, Issue 6180, pp. 172-177, 2014.
- [2] N. Franceschini, “Small Brains, Smart Machines: From Fly Vision to Robot Vision and Back Again,” Proc. of IEEE, Vol.102, Issue 5, pp. 751-781, 2014.
- [3] B. Hassenstein and W. Reichardt, “Systemtheoretische Analyse der Zeit-, Reihenfolgen- und Vorzeichenauswertung bei der Bewegungsperzeption des Rüsselkäfers Chlorophanus,” Zeitschrift für Naturforschung B, Vol.11, Nos.9-10, pp. 513-524, 1952.
- [4] H. G. Meyer, O. J. N. Bertrand, J. Paskarbeit, J. P. Lindemann, A. Schneider, and M. Egelhaaf, “A Bio-Inspired Model for Visual Collision Avoidance on a Hexapod Walking Robot,” Proc. of 5th Int. Conf. on Biomimetic and Biohybrid Systems, Living Machines, pp. 167-178, 2016.
- [5] J. R. Serres and S. Viollet, “Insect-inspired vision for autonomous vehicles,” Current Opinion in Insect Science, Vol.30, pp. 46-51, 2018.
- [6] H. B. Barlow, R. M. Hill, and W. R. Levick, “Retinal ganglion cells responding selectively to direction and speed of image motion in the rabbit,” The J. of Physiology, Vol.173, No.3, pp. 377-407, 1964.
- [7] H. B. Barlow and W. R. Levick, “The mechanism of directionally selective units in rabbit’s retina,” The J. of Physiology, Vol.178, No.3, pp. 477-504, 1964.
- [8] M. Joesch, B. Schnell, S. V. Raghu, D. F. Reiff, and A. Borst, “ON and OFF pathways in Drosophila motion vision,” Nature, Vol.468, pp. 300-304, 2010.
- [9] H. Eichner, M. Joesch, B. Schnell, D. F. Reiff, and A. Borst, “Internal Structure of the Fly Elementary Motion Detector,” Neuron, Vol.70, Issue 6, pp. 1155-1164, 2011.
- [10] M. S. Maisak, J. Haag, G. Ammer, E. Serbe, M. Meier, A. Leonhardt, T. Schilling, A. Bahl, G. M. Rubin, A. Nern, B. J. Dickson, D. F. Reiff, E. Hopp, and A. Borst, “A directional tuning map of Drosophila elementary motion detectors,” Nature, Vol.500, pp. 212-216, 2013.
- [11] S. Takemura, A. Bharioke, Z. Lu, A. Nern, S. Vitaladevuni, P. K. Rivlin, W. T. Katz, D. J. Olbris, S. M. Plaza, P. Winston, T. Zhao, J. A. Horne, R. D. Fetter, S. Takemura, K. Blazek, L.-A. Chang, O. Ogundeyi, M. A. Saunders, V. Shapiro, C. Sigmund, G. M. Rubin, L. K. Scheffer, I. A. Meinertzhagen, and D. B. Chklovskii, “A visual motion detection circuit suggested by Drosophila connectomics,” Nature, Vol.500, No.7461, pp. 175-181, 2013.
- [12] K. Shinomiya, T. Karuppudurai, T.-Y. Lin, Z. Lu, C.-H. Lee, and I. A. Meinertzhagen, “Candidate Neural Substrates for OFF-Edge Motion Detection in Drosophila,” Current Biology, Vol.24, Issue 10, pp. 1062-1070, 2014.
- [13] J. Haag, A. Arenz, E. Serbe, F. Gabbiani, and A. Borst, “Complementary mechanisms create direction selectivity in the fly,” eLife, Vol.5, Article No.e17421, 2016.
- [14] J. Haag, A. Mishra, and A. Borst, “A common directional tuning mechanism of Drosophila motion-sensing neurons in the ON and in the OFF pathway,” eLife, Vol.6, Article No.e29044, 2017.
- [15] A. Borst, “A biophysical mechanism for preferred direction enhancement in fly motion vision,” PLoS Comput. Biol., Vol.14, No.6, Article No.e1006240, 2018.
- [16] E. Gruntman, S. Romani, and M. B. Reiser, “Simple integration of fast excitation and offset, delayed inhibition computes directional selectivity in Drosophila,” Nature Neuroscience, Vol.21, pp. 250-257, 2018.
- [17] F. D. Tschopp, M. B. Reiser, and S. C. Turaga, “A connectome based hexagonal lattice convolutional network model of the Drosophila visual system,” arXiv:1806.04793, 2018.
- [18] A. Arenz, M. S. Drews, F. G. Richter, G. Ammer, and A. Borst, “The Temporal Tuning of the Drosophila Motion Detectors Is Determined by the Dynamics of Their Input Elements,” Current Biology, Vol.27, Issue 7, pp. 929-944, 2017.
- [19] S. Takemura, A. Nern, D. B. Chklovskii, L. K. Scheffer, G. M. Rubin, and I. A. Meinertzhagen, “The comprehensive connectome of a neural substrate for ‘on’ motion detection in Drosophila,” elife, Vol.6, Article No.e24394, 2017.
- [20] J. A. Strother, S.-T. Wu, A. M. Wong, A. Nern, E. M. Rogers, J. Q. Le, G. M. Rubin, and M. B. Reiser, “The Emergence of Directional Selectivity in the Visual Motion Pathway of Drosophila,” Neuron, Vol.94, No.1, pp. 168-182.e10, 2017.
- [21] M. L. Hines and N. T. Carnevale, “The neuron simulation environment,” Neural Computation, Vol.9, No.6, pp. 1179-1209, 1997.
- [22] A. Kolodziejczyk, X. Sun, I. Meinertzhagen, and D. R. Nässel, “Glutamate, GABA and acetylcholine signaling components in the lamina of the Drosophila visual system,” PLoS ONE, Vol.3, No.5, Article No.e2110, 2008.
- [23] J. E. Niven, M. Vähäsöyrinki, M. Kauranen, R. C. Hardir, M. Juusola, and M. Weckström, “The contribution of Shaker K+ channels to the information capacity of Drosophila photoreceptors,” Nature, Vol.421, pp. 630-634, 2003.
- [24] J. K. Douglass and N. J. Strausfeld, “Visual Motion Detection Circuits in Flies: Peripheral Motion Computation by Identified Small-Field Retinotopic Neurons,” J. of Neuroscience, Vol.15, No.8, pp. 5596-5611, 1995.
- [25] S. B. Laughlin, “Coding Efficiency and Design in Visual Processing,” D. G. Stavenga and R. C. Hardie (Eds.), “Facets of vision,” Springer, pp. 213-234, 1989.
- [26] C. Gilbert and N. J. Strausfeld, “Small-field neurons associated with oculomotor and optomotor control in muscoid flies: Functional organization,” The J. of Comparative Neurology, Vol.316, No.1, pp. 72-86, 1992.
- [27] H. H. Yang and T. R. Clandinin, “Elementary Motion Detection in Drosophila: Algorithms and Mechanisms,” Annual Review of Vision Science, Vol.4, pp. 143-163, 2018.
- [28] J. Rusanen and M. Weckström, “Frequency-selective transmission of graded signals in large monopolar neurons of blowfly Calliphora vicina compound eye,” J. Neurophysiol., Vol.115, No.4, pp. 2052-2064, 2016.
- [29] C. Morris and H. Lecar, “Voltage Oscillations in the barnacle giant muscle fiber,” Biophys. J., Vol.35, No.1, pp. 193-213, 1981.
- [30] S.-Y. Takemura, C. S. Xu, Z. Lu, P. K. Rivlin, T. Parag, D. J. Olbris, S. Plaza, T. Zhao, W. T. Katz, L. Umayam, C. Weaver, H. F. Hess, J. A. Horne, J. Nunez-Iglesias, R. Aniceto, L. Chang, S. Lauchie, A. Nasca, O. Ogundeyi, C. Sigmund, S. Takemura, J. Tran, C. Langille, K. L. Lacheur, S. McLin, A. Shinomiya, D. B Chklovskii, I. A. Meinertzhagen, and L. K. Scheffer, “Synaptic circuits and their variations within different columns in the visual system of Drosophila,” Proc. of the National Academy of Sciences, Vol.112, No.44, pp. 13711-13716, 2015.
- [31] F. P. Davis, A. Nern, S. Picard, M. B. Reiser, G. M. Rubin, S. R. Eddy, and G. L. Henry, “A genetic, genomic, and computational resource for exploring neural circuit function,” eLife, Vol.9, No.9, Article No.e50901, 2020.
- [32] J. A. Strother, S.-T. Wu, E. M. Rogers, J. L. M. Eliason, A. M. Wong, A. Nern, and M. B. Reiser, “Behavioral state modulates the ON visual motion pathway of Drosophila,” PNAS, Vol.115, No.1, pp. E102-E111, 2017.
- [33] X.-J. Wang, Y. Liu, M. V. Sanchez-Vives, and D. A. McCormick, “Adaptation and Temporal Decorrelation by Single Neurons in the Primary Visual Cortex,” Neurophysiology, Vol.89, Issue 6, pp. 3279-3293, 2003.
- [34] M. V. Sanchez-Vives, L. G. Nowak, and D. A. McCormick, “Cellular Mechanisms of Long-Lasting Adaptation in Visual Cortical Neurons in Vitro,” J. of Neuroscience, Vol.20, No.11, pp. 4286-4299, 2000.
- [35] D. Pérez-González and M. S. Malmierca, “Adaptation in the auditory system: an overview,” Frontiers in Integrative Neuroscience, Vol.8, p. 19, 2014.
- [36] K. Friston, “The free-energy principle: a unified brain theory?,” Nature Reviews Neuroscience, Vol.11, pp. 127-138, 2010.
- [37] T. Kazawa, T. Fukuda, A. Goto, D. Miyamoto, S. S. Haupt, and R. Kanzaki, “Adapting CMA-ES for the Massively Parallelized Simulation of Neurons and Neural Circuits,” DEStech Trans. on Engineering and Technology Research, 2019.
- [38] T. Schoepe, E. Janotte, M. B. Milde, O. J. N. Bertrand, M. Egelhaaf, and E. Chicca, “Finding the gap: Neuromorphic motion vision in cluttered environments,” arXiv:2102.08417, 2021.
- [39] C. D. Schuman, S. R. Kulkarni, M. Parsa, J. P. Mitchell, P. Date, and B. Kay, “Opportunities for neuromorphic computing algorithms and applications,” Nature Computational Science, Vol.2, pp. 10-19, 2022.
- [40] Y. Hosoda, R. Sawahashi, N. Machinaka, R. Yamazaki, Y. Sadakuni, K. Onda, R. Kusakari, M. Kimba, T. Oishi, and Y. Kuroda, “Robust Road-Following Navigation System with a Simple Map,” J. Robot. Mechatron., Vol.30, No.4, pp. 552-562, 2018.
- [41] B. Cheng and X. Deng, “A Neural Adaptive Controller in Flapping Flight,” J. Robot. Mechatron., Vol.24, No.4, pp. 602-611, 2012.
- [42] K. Shinomiya, G. Huang, Z. Lu, T. Parag, C. S. Xu, R. Aniceto, N. Ansari, N. Cheatham, S. Lauchie, E. Neace, O. Ogundeyi, C. Ordish, D. Peel, A. Shinomiya, C. Smith, and S. Takemura, “Comparisons between the ON- and OFF-edge motion pathways in the Drosophila brain,” eLife, Vol.8, Article No.e40025, 2019.
- [43] J. R. Kohn, J. P. Portes, M. P. Christenson, L. F. Abbott, and R. Behnia, “Flexible filtering by neural inputs supports motion computation across states and stimuli,” Current Biology, Vol.31, Issue 23, pp. 5249-5260.e5, 2021.
- [44] O. Mano, M. S. Creamer, B. A. Badwan, and D. A. Clark, “Predicting individual neuron responses with anatomically constrained task optimization,” Current Biology, Vol.31, Issue 18, pp. 4062-4075.e4, 2021.
This article is published under a Creative Commons Attribution-NoDerivatives 4.0 Internationa License.