Paper:
Active Passive Nature of Assistive Wearable Gait Augment Suit for Enhanced Mobility
Chetan Thakur*, Kazunori Ogawa*,**, and Yuichi Kurita*,***
*Graduate School of Engineering, Hiroshima University
1-4-1 Kagamiyama, Higashihiroshima, Hiroshima 739-8527, Japan
**Daiya Industries Co., Ltd.
1125 Koshinden, Minami-ku, Okayama-shi, Okayama 701-0203, Japan
***PRESTO (Sakigake), Japan Society and Technology Agency (JST)
7 Gobancho, Chiyoda-ku, Tokyo 102-0076, Japan
In this paper we discuss the active and passive nature of the assistive wearable gait augment suit (AWGAS). AWGAS is a soft, wearable, lightweight, and assists walking gait by reducing muscle activation during walking. It augments walking by reducing the muscle activation of the posterior and anterior muscles of the lower limb. The suit uses pneumatic gel muscles (PGM), foot sensors for gait detection, and pneumatic valves to control the air pressure. The assistive force is provided using the motion in loop feedforward control loop using foot sensors in shoes. PGMs are actuated with the help of pneumatic valves and portable air tanks. The elastic nature of the PGM allows AWGAS to assist walking in the absence of the air supply which makes AWGAS both active and passive walking assist suit. To evaluate the active and passive nature of the AWGAS, we experimented to measure surface EMG (sEMG) of the lower limb muscles. sEMG was recorded for unassisted walking, i.e., without the suit, passive assisted walking, i.e., wearing the suit with no air supply and active assisted walking, i.e., wearing the suit with air supply set at 60 kPa. The results shows reduction in the muscle activity for both passive and active assisted walking as compared to unassisted walking. The pilot trials of the AWGAS were conducted in collaboration with local farmers in the Hiroshima prefecture in Japan where feedback received is complementing the results obtained during the experiments.
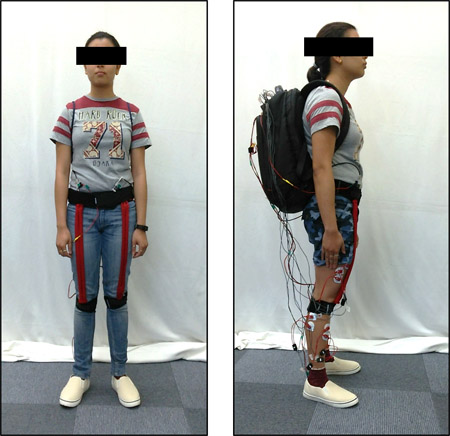
Low powered and lightweight AWGAS with active and passive assistive nature
- [1] K. Suzuki, G. Mito, H. Kawamoto, Y. Hasegawa, and Y. Sankai, “Intention-Based Walking Support for Paraplegia Patients with Robot Suit HAL,” Adv. Robot., Vol.21, No.12, pp. 1441-1469, 2007.
- [2] S. Toyama and G. Yamamoto, “Development of wearable-agri-robot-Mechanism for agricultural work,” 2009 IEEE/RSJ Int. Conf. on Intelligent Robots and Systems (IROS 2009), pp. 5801-5806, 2009.
- [3] Y. Ikeuchi, J. Ashihara, Y. Hiki, H. Kudoh, and T. Noda, “Walking assist device with bodyweight support system,” 2009 IEEE/RSJ Int. Conf. on Intelligent Robots and Systems (IROS 2009), pp. 4073-4079, 2009.
- [4] P. Malcolm, W. Derave, S. Galle, and D. De Clercq, “A Simple Exoskeleton That Assists Plantarflexion Can Reduce the Metabolic Cost of Human Walking,” PLoS One, Vol.8, No.2, e56137, 2013.
- [5] M. Ishii, K. Yamamoto, and K. Hyodo, “Stand-Alone Wearable Power Assist Suit – Development and Availability,” J. Robot. Mechatron., Vol.17, No.5, pp. 575-583, 2005.
- [6] Y. Naruoka, N. Hiramitsu, and Y. Mitsuya, “A study of power-assist technology to reduce body burden during loading and unloading operations by support of knee joint motion,” J. Robot. Mechatron., Vol.28, No.6, pp. 949-957, 2016.
- [7] K. Sano, E. Yagi, and M. Sato, “Development of a Wearable Assist Suit for Walking and Lifting-Up Motion Using Electric Motors,” J. Robot. Mechatron., Vol.25, No.6, pp. 923-930, 2013.
- [8] T. Noritsugu, D. Sasaki, M. Kameda, A. Fukunaga, and M. Takaiwa, “Wearable power assist device for standing upmotion using pneumatic rubber artificial muscles,” J. Robot. Mechatron., Vol.19, No.6, pp. 619-628, 2007.
- [9] B. T. Quinlivan et al., “Assistance magnitude versus metabolic cost reductions for a tethered multiarticular soft exosuit,” Science Robotics, Vol.2, No.2, eaah4416, 2017.
- [10] A. T. Asbeck, K. Schmidt, and C. J. Walsh, “Soft exosuit for hip assistance,” Rob. Auton. Syst., Vol.73, pp. 102-110, 2015.
- [11] K. Schmidt et al., “The myosuit: Bi-articular anti-gravity exosuit that reduces hip extensor activity in sitting transfers,” Front. Neurorobot., Vol.11, p. 116, 2017.
- [12] A. T. Asbeck, S. M. M. De Rossi, K. G. Holt, and C. J. Walsh, “A biologically inspired soft exosuit for walking assistance,” Int. J. Rob. Res., Vol.34, No.6, pp. 744-762, 2015.
- [13] K. Ogawa, C. Thakur, T. Ikeda, T. Tsuji, and Y. Kurita, “Development of a pneumatic artificial muscle driven by low pressure and its application to the unplugged powered suit,” Adv. Robot., Vol.31, No.21, pp. 1135-1143, 2017.
- [14] F. Daerden and D. Lefeber, “Pneumatic artificial muscles: actuators for robotics and automation,” Eur. J. Mech. Eng., Vol.47, No.1, p. 1021, 2000.
- [15] J. Perry and J. Burnfield, “GAIT Normal and Pathological Function,” J. Sports Sci. Med., Vol.9, No.2, p. 551, 2010.
- [16] S. Chen, J. Lach, B. Lo, and G. Z. Yang, “Toward Pervasive Gait Analysis With Wearable Sensors: A Systematic Review,” IEEE J. Biomed. Heal. Informatics, Vol.20, No.6, pp. 1521-1537, 2016.
- [17] Y. Long, Z.-J. Du, W.-D. Wang, and W. Dong, “Human motion intent learning based motion assistance control for a wearable exoskeleton,” Robot. Comput. Integr. Manuf., Vol.49, pp. 317-327, 2018.
- [18] J. Taborri, E. Palermo, S. Rossi, and P. Cappa, “Gait partitioning methods: A systematic review,” Sensors, Vol.16, No.1, pii: E66, 2016.
- [19] C. Kirtley, M. W. Whittle, and R. J. Jefferson, “Influence of walking speed on gait parameters,” J. Biomed. Eng., Vol.7, No.4, pp. 282-288, 1985.
- [20] T. P. Andriacchi, J. A. Ogle, and J. O. Galante, “Walking speed as a basis for normal and abnormal gait measurements,” J. Biomech., Vol.10, No.4, pp. 261-268, 1977.
- [21] S. Oh, E. Baek, S. K. Song, S. Mohammed, D. Jeon, and K. Kong, “A generalized control framework of assistive controllers and its application to lower limb exoskeletons,” Rob. Auton. Syst., Vol.73, pp. 68-77, 2015.
- [22] R. W. Kressig and O. Beauchet, “Guidelines for clinical applications of spatio-temporal gait analysis in older adults,” Aging Clin. Exp. Res., Vol.18, No.2, pp. 174-176, 2006.
- [23] N. Aliman, R. Ramli, and S. M. M. Haris, “Design and development of lower limb exoskeletons: A survey,” Rob. Auton. Syst., Vol.95, pp. 102-116, 2017.
- [24] A. T. Asbeck, S. M. M. De Rossi, I. Galiana, Y. Ding, and C. J. Walsh, “Stronger, smarter, softer: Next-generation wearable robots,” IEEE Robot. Autom. Mag., Vol.21, No.4, pp. 22-33, 2014.
- [25] K. Kong and M. Tomizuka, “Smooth and continuous human gait phase detection based on foot pressure patterns,” Proc. IEEE Int. Conf. Robot. Autom., pp. 3678-3683, 2008.
- [26] K. Kong, J. Bae, and M. Tomizuka, “Impedance compensation of flexible joint actuator for ideal force mode control,” IFAC Proc. Volumes, Vol.41, No.2, pp. 2442-2447, 2008.
- [27] S. H. Collins, M. B. Wiggin, and G. S. Sawicki, “Reducing the energy cost of human walking using an unpowered exoskeleton,” Nature, Vol.522, No.7555, pp. 212-215, 2015.
- [28] C. P. Chou and B. Hannaford, “Measurement and modeling of McKibben pneumatic artificial muscles,” IEEE Trans. Robot. Autom., Vol.12, No.1, pp. 90-102, 1996.
This article is published under a Creative Commons Attribution-NoDerivatives 4.0 Internationa License.