Paper:
A Motor Adaptation Model Assuming Update of Internal Model in the Motor Cortex
Sho Furubayashi, Takahiro Hasegawa, and Eizo Miyashita
School of Life Science and Technology, Tokyo Institute of Technology
4259 Nagatsuta-cho, Midori-ku, Yokohama, Kanagawa 226-8501, Japan
When considering the human motor-adaptation mechanism from the perspective of the motor control theory, updating the internal model constitutes a critical component. The learning curve at each trial of motion can be explained by a state-space model; however, the model cannot reproduce the time-series data for the hand’s position, velocity, and acceleration (motion profiles). There is no internal model-updating rule for optimal feedback control, a plausible model for reproducing motion profiles. In this paper, we propose an adaptation model that incorporates an internal model-updating rule which modeled after Hebb’s rule into optimal feedback control. Also, we examine the neural substrate of the internal model. To validate the proposed adaptation model, we conducted behavioral experiments with humans that reflected changes in the internal model and reproduced the changes in the internal model as well as the motion profiles using the proposed adaptation model. In addition, we analyzed the data for a visuomotor rotation task performed by a monkey and checked for changes in the output characteristics of neurons in the motor cortex before and after adaptation. According to the above-mentioned validation and analysis results, the motor cortex constitutes the neural substrate of the internal model.
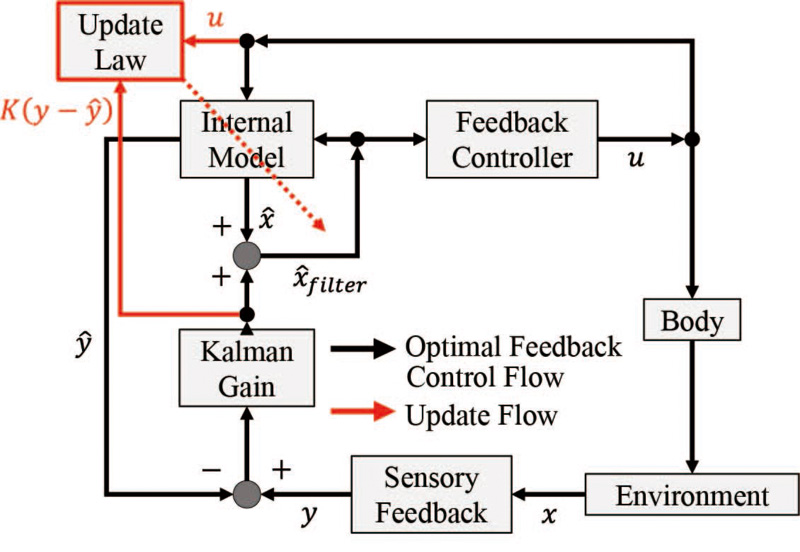
Flow of the adaptation model
- [1] V. S. Huang and J. W. Krakauer, “Robotic neurorehabilitation: a computational motor learning perspective,” J. of NeuroEngineering and Rehabilitation, Vol.6, No.5, doi: 10.1186/1743-0003-6-5, 2009.
- [2] E. Dayan and L. G. Cohen, “Neuroplasticity Subserving Motor Skill Learning,” Neuron, Vol.72, No.3, pp. 443-454, doi: 10.1016/j.neuron.2011.10.008, 2011.
- [3] J. W. Krakauer, A. M. Hadjiosif, J. Xu, A. L. Wong, and A. M. Haith, “Motor Learning,” Compr. Physiol., Vol.9, No.2, pp. 613-663, doi: 10.1002/cphy.c170043, 2019.
- [4] G. Anobile, N. Domenici, I. Togoli, D. Burr, and R. Arrighi, “Distortions of visual time induced by motor adaptation,” J. of Experimental Psychology: General, Vol.149, No.7, pp. 1333-1343, doi: 10.1037/xge0000709, 2020.
- [5] L. Johannsmeier, M. Gerchow, and S. Haddadin, “A Framework for Robot Manipulation: Skill Formalism, Meta Learning and Adaptive Control,” 2019 Int. Conf. on Robotics and Automation (ICRA), pp. 5844-5850, doi: 10.1109/ICRA.2019.8793542, May 2019.
- [6] H. Huang, C. Yang, and C. L. P. Chen, “Optimal Robot–Environment Interaction Under Broad Fuzzy Neural Adaptive Control,” IEEE Trans. on Cybernetics, Vol.51, No.7, pp. 3824-3835, doi: 10.1109/TCYB.2020.2998984, 2021.
- [7] R. C. Miall and D. M. Wolpert, “Forward Models for Physiological Motor Control,” Neural Networks, Vol.9, No.8, pp. 1265-1279, doi: 10.1016/S0893-6080(96)00035-4, 1996.
- [8] D. M. Wolpert, R. C. Miall, and M. Kawato, “Internal models in the cerebellum,” Trends in Cognitive Sciences, Vol.2, No.9, pp. 338-347, doi: 10.1016/S1364-6613(98)01221-2, 1998.
- [9] J. Izawa and R. Shadmehr, “Learning from Sensory and Reward Prediction Errors during Motor Adaptation,” PLOS Computational Biology, Vol.7, No.3, e1002012, doi: 10.1371/journal.pcbi.1002012, 2011.
- [10] K. Ito, M. Doi, and T. Kondo, “Feed-Forward Adaptation to a Varying Dynamic Environment During Reaching Movements,” J. Robot. Mechatron., Vol.19, No.4, pp. 474-481, doi: 10.20965/jrm.2007.p0474, 2007.
- [11] M. Kawato, “Internal models for motor control and trajectory planning,” Current Opinion in Neurobiology, Vol.9, No.6, pp. 718-727, doi: 10.1016/S0959-4388(99)00028-8, 1999.
- [12] V. Dietz, M. Discher, and M. Trippel, “Task-dependent modulation of short- and long-latency electromyographic responses in upper limb muscles,” Electroencephalography and Clinical Neurophysiology/Evoked Potentials Section, Vol.93, No.1, pp. 49-56, doi: 10.1016/0168-5597(94)90091-4, 1994.
- [13] G. N. Lewis, C. D. MacKinnon, and E. J. Perreault, “The effect of task instruction on the excitability of spinal and supraspinal reflex pathways projecting to the biceps muscle,” Exp. Brain Res., Vol.174, No.3, pp. 413-425, doi: 10.1007/s00221-006-0475-x, 2006.
- [14] D. W. Franklin and D. M. Wolpert, “Specificity of Reflex Adaptation for Task-Relevant Variability,” J. Neurosci., Vol.28, No.52, pp. 14165-14175, doi: 10.1523/JNEUROSCI.4406-08.2008, 2008.
- [15] M. A. Smith and R. Shadmehr, “Intact Ability to Learn Internal Models of Arm Dynamics in Huntington’s Disease But Not Cerebellar Degeneration,” J. of Neurophysiology, Vol.93, No.5, pp. 2809-2821, doi: 10.1152/jn.00943.2004, 2005.
- [16] P. Mazzoni and J. W. Krakauer, “An Implicit Plan Overrides an Explicit Strategy during Visuomotor Adaptation,” J. Neurosci., Vol.26, No.14, pp. 3642-3645, doi: 10.1523/JNEUROSCI.5317-05.2006, 2006.
- [17] J. R. Morehead, J. A. Taylor, D. E. Parvin, and R. B. Ivry, “Characteristics of Implicit Sensorimotor Adaptation Revealed by Task-irrelevant Clamped Feedback,” J. of Cognitive Neuroscience, Vol.29, No.6, pp. 1061-1074, doi: 10.1162/jocn_a_01108, 2017.
- [18] J. A. Taylor, J. W. Krakauer, and R. B. Ivry, “Explicit and Implicit Contributions to Learning in a Sensorimotor Adaptation Task,” J. Neurosci., Vol.34, No.8, pp. 3023-3032, doi: 10.1523/JNEUROSCI.3619-13.2014, 2014.
- [19] J. R. Morehead, S. E. Qasim, M. J. Crossley, and R. Ivry, “Savings upon Re-Aiming in Visuomotor Adaptation,” J. Neurosci., Vol.35, No.42, pp. 14386-14396, doi: 10.1523/JNEUROSCI.1046-15.2015, 2015.
- [20] S. D. McDougle, K. M. Bond, and J. A. Taylor, “Explicit and Implicit Processes Constitute the Fast and Slow Processes of Sensorimotor Learning,” J. Neurosci., Vol.35, No.26, pp. 9568-9579, doi: 10.1523/JNEUROSCI.5061-14.2015, 2015.
- [21] M. A. Smith, A. Ghazizadeh, and R. Shadmehr, “Interacting Adaptive Processes with Different Timescales Underlie Short-Term Motor Learning,” PLOS Biology, Vol.4, No.6, e179, doi: 10.1371/journal.pbio.0040179, 2006.
- [22] E. Todorov and M. I. Jordan, “Optimal feedback control as a theory of motor coordination,” Nat. Neurosci., Vol.5, No.11, pp. 1226-1235, doi: 10.1038/nn963, 2002.
- [23] J. Izawa and R. Shadmehr, “On-Line Processing of Uncertain Information in Visuomotor Control,” J. Neurosci., Vol.28, No.44, pp. 11360-11368, doi: 10.1523/JNEUROSCI.3063-08.2008, 2008.
- [24] Y. Ueyama and E. Miyashita, “Optimal Feedback Control for Predicting Dynamic Stiffness During Arm Movement,” IEEE Trans. on Industrial Electronics, Vol.61, No.2, pp. 1044-1052, doi: 10.1109/TIE.2013.2273473, 2014.
- [25] H. Tanaka, T. Ishikawa, J. Lee, and S. Kakei, “The Cerebro-Cerebellum as a Locus of Forward Model: A Review,” Frontiers in Systems Neuroscience, Vol.14, No.19, doi: 10.3389/fnsys.2020.00019, 2020.
- [26] E. Miyashita and Y. Sakaguchi, “State Variables of the Arm May Be Encoded by Single Neuron Activity in the Monkey Motor Cortex,” IEEE Trans. on Industrial Electronics, Vol.63, No.3, pp. 1943-1952, doi: 10.1109/TIE.2015.2504579, 2016.
- [27] S. H. Scott, “Optimal feedback control and the neural basis of volitional motor control,” Nature Reviews Neuroscience, Vol.5, No.7, pp. 532-545, doi: 10.1038/nrn1427, 2004.
- [28] C. Pavlides, E. Miyashita, and H. Asanuma, “Projection from the sensory to the motor cortex is important in learning motor skills in the monkey,” J. of Neurophysiology, Vol.70, No.2, pp. 733-741, doi: 10.1152/jn.1993.70.2.733, 1993.
- [29] A. Keller, E. Miyashita, and H. Asanuma, “Minimal stimulus parameters and the effects of hyperpolarization on the induction of long-term potentiation in the cat motor cortex,” Exp. Brain Res., Vol.87, No.2, pp. 295-302, doi: 10.1007/BF00231846, 1991.
- [30] R. Nudo, G. Milliken, W. Jenkins, and M. Merzenich, “Use-dependent alterations of movement representations in primary motor cortex of adult squirrel monkeys,” J. Neurosci., Vol.16, No.2, pp. 785-807, doi: 10.1523/JNEUROSCI.16-02-00785.1996, 1996.
- [31] D. H. Brainard, “The Psychophysics Toolbox,” Spat. Vis., Vol.10, No.4, pp. 433-436, 1997.
- [32] D. G. Pelli, “The VideoToolbox software for visual psychophysics: transforming numbers into movies,” Spat. Vis., Vol.10, No.4, pp. 437-442, 1997.
- [33] M. Kleiner, D. Brainard, D. Pelli, A. Ingling, R. Murray, and C. Broussard, “What’s new in psychtoolbox-3,” Perception, Vol.36, No.14, pp. 1-16, 2007.
- [34] D. J. Bennett, “Electromyographic responses to constant position errors imposed during voluntary elbow joint movement in human,” Experimental Brain Research, Vol.95, No.3, pp. 499-508, doi: 10.1007/BF00227143, 1993.
- [35] D. C. Knill and A. Pouget, “The Bayesian brain: the role of uncertainty in neural coding and computation,” Trends in Neurosciences, Vol.27, No.12, pp. 712-719, doi: 10.1016/j.tins.2004.10.007, 2004.
- [36] S. Murakami and E. Miyashita, “Effects of movement variability of dominant and nondominant arm on adaptation to visual disturbances,” J. Physiol. Sci., Vol.63, pp. S285-S285, 2013.
- [37] A. Battaglia-Mayer and R. Caminiti, “Corticocortical Systems Underlying High-Order Motor Control,” J. Neurosci., Vol.39, No.23, pp. 4404-4421, doi: 10.1523/JNEUROSCI.2094-18.2019, 2019.
- [38] K. Kultas-Ilinsky, E. Sivan-Loukianova, and I. A. Ilinsky, “Reevaluation of the primary motor cortex connections with the thalamus in primates,” J. of Comparative Neurology, Vol.457, No.2, pp. 133-158, doi: 10.1002/cne.10539, 2003.
- [39] A. Iriki, C. Pavlides, A. Keller, and H. Asanuma, “Long-term potentiation in the motor cortex,” Science, Vol.245, No.4924, pp. 1385-1387, doi: 10.1126/science.2551038, 1989.
- [40] A. Iriki, C. Pavlides, A. Keller, and H. Asanuma, “Long-term potentiation of thalamic input to the motor cortex induced by coactivation of thalamocortical and corticocortical afferents,” J. of Neurophysiology, Vol.65, No.6, pp. 1435-1441, doi: 10.1152/jn.1991.65.6.1435, 1991.
- [41] J. Diedrichsen, Y. Hashambhoy, T. Rane, and R. Shadmehr, “Neural Correlates of Reach Errors,” J. Neurosci., Vol.25, No.43, pp. 9919-9931, doi: 10.1523/JNEUROSCI.1874-05.2005, 2005.
- [42] M. Inoue and S. Kitazawa, “Motor Error in Parietal Area 5 and Target Error in Area 7 Drive Distinctive Adaptation in Reaching,” Current Biology, Vol.28, No.14, pp. 2250-2262, doi: 10.1016/j.cub.2018.05.056, 2018.
- [43] E. Miyashita, M. Komatsu, Y. Cheng, H. Tsukada, and H. Once, “At least two different neural circuits are involved in visuo-motor adaptation: a PET study,” J. Physiol. Sci., Vol.59, pp. 426-426, 2009.
This article is published under a Creative Commons Attribution-NoDerivatives 4.0 Internationa License.